Hong Kong Med J 2022 Apr;28(2):140–51 | Epub 8 Apr 2022
© Hong Kong Academy of Medicine. CC BY-NC-ND 4.0
ORIGINAL ARTICLE
Antibiogram data from private hospitals in Hong
Kong: 6-year retrospective study
Leo Lui, MB, BS, FHKAM (Pathology); LC Wong, MSc; H Chen, MB, BS, FHKAM (Community Medicine); Raymond WH Yung, MB, BS, FHKAM (Pathology); for The Working Group of Collaboration between
CHP and Private Hospitals on Safe Use of Antibiotics and Infection Control
1 Infection Control Branch, Centre for Health Protection, Hong Kong SAR Government, Hong Kong
2 Department of Pathology (Clinical Microbiology), Hong Kong Sanatorium & Hospital, Hong Kong
Corresponding author: Dr Leo Lui (leo_lui@dh.gov.hk)

Abstract
Introduction: The surveillance of antibiotic
resistance is critical for the establishment of
effective control strategies. The antibiotic resistance
situations in private hospitals in Hong Kong have
not been systematically described. The objective
of the study was to analyse antibiogram data from
private hospitals and describe the temporal trends of
non-susceptibility percentages in this setting.
Methods: This retrospective descriptive study used
antibiogram data from all private hospitals in Hong
Kong that had been collected annually for 6 years
(2014-2019). Data on six targeted bacteria and their
corresponding multidrug-resistant organisms were
included.
Results: The non-susceptibility percentages of
isolates remained stable or decreased during the
study period: methicillin-resistant Staphylococcus
aureus had a stable prevalence of approximately
20%; extended-spectrum beta-lactamase-producing
Escherichia coli and Klebsiella species had stable
prevalences of 20% to 30% and 10% to 20%,
respectively; multidrug-resistant Acinetobacter
species had prevalences of approximately 2% to 8%,
which decreased over time; multidrug-resistant
Pseudomonas aeruginosa had prevalences of 0.0%
to 0.3%; Streptococcus pneumoniae penicillin and
macrolide non-susceptibility percentages were 2% to 9% and 71% to 79%, respectively. These values
generally were comparable with findings from
public hospitals and Residential Care Homes for the
Elderly in Hong Kong. However, the prevalences of
carbapenem-resistant Enterobacteriaceae, which are
increasing in Hong Kong and other nations, were
also increasing in our dataset despite their currently
low values (<1% for Escherichia coli and <2% for
Klebsiella species).
Conclusion: The antibiotic resistance landscape
among private hospitals in Hong Kong is satisfactory
overall; there remains a need for surveillance,
antibiotic stewardship, and other infection control
measures.
New knowledge added by this study
- This report of antibiotic resistance prevalence includes 6 years of data from all private hospitals in Hong Kong.
- The prevalences of methicillin-resistant Staphylococcus aureus and extended-spectrum beta-lactamase-producing Escherichia coli and Klebsiella species were moderate but stable (approximately 20%).
- The prevalences of multidrug-resistant Acinetobacter species (approximately 2%-8%) and multidrug-resistant Pseudomonas aeruginosa (0%-0.3%) were low.
- Antibiogram data can be used to monitor antibiotic resistance trends, which may help to guide empirical treatment and assess the effectiveness of infection control measures.
- The lower prevalences of multidrug-resistant organisms (MDROs) in private hospitals (compared with public hospitals) may be related to the presence of additional staff members and the use of a strict MDRO carrier isolation policy.
Introduction
Surveillance is a critical aspect of antibiotic resistance
control. Susceptibility data periodically collected
from patients can be used to construct antibiograms
for monitoring of resistance trends and guidance of
empirical treatment.1
The Working Group of Collaboration between
the Centre for Health Protection (CHP) and Private
Hospitals on Safe Use of Antibiotics and Infection
Control (the “Working Group”) was established to
exchange information regarding infection prevention
and to promote safe antibiotic use. Members included the Infection Control Branch of the CHP
and the Hong Kong Private Hospitals Association.
Each year, the Infection Control Branch collected
from and shared the susceptibility data with private
hospitals. The resulting antibiograms were uploaded
to the CHP website.2
Hong Kong runs a dual-track healthcare
system, in which the private sector complements the
public system with a range of general and specialist
services. In accordance with the market-set price
principle, private hospitals and out-patient clinics
establish their charges based on the costs of services
provided. Although private sector expenses can be
severalfold higher than the expenses of their public
counterparts, services provided by the private sector
are often considered more flexible and personalised;
thus, they are more appealing to individuals with
the ability and interest in payment for such services.
In 2018, private hospitals provided approximately
5000 beds for >370 000 in-patients, which
constituted approximately 17% of all in-patients in
Hong Kong.3 4
The objective of this study was to analyse the
antibiogram data collected from private hospitals
in Hong Kong by describing the temporal trends of
non-susceptibility percentages in this setting. The
results may provide insights regarding the current
level of antibiotic resistance in private hospitals, in
comparison with other settings; they may guide the
establishment of future surveillance methods.
Methods
Private hospitals included
The surveillance data submitted by all 12 private
hospitals during the period from 2014 to 2019
were included in this study. Please refer to the
Acknowledgement for the membership of the
Working Group and their affiliated hospitals/institutions.
Targeted bacteria
Considering the antibiotic resistance situations in
Hong Kong and other countries, as well as the health
effects of various bacterial species, members of the
Working Group agreed upon six targeted bacteria
for the annual submission of antibiotic susceptibility
testing (AST) results, including: Staphylococcus
aureus, Escherichia coli, Klebsiella spp, Pseudomonas
aeruginosa, Acinetobacter spp, and Streptococcus
pneumoniae (since 2015).
Multidrug-resistant organisms
Resistant strains of the targeted bacteria can cause
increased morbidity and mortality because of
limited treatment options. International health
authorities have set clear priorities in their efforts to
control multidrug-resistant organisms (MDROs).5 6
The definitions for MDROs used in this study were
as follows: methicillin-resistant S aureus (MRSA)
demonstrated resistance to methicillin, oxacillin,
cefoxitin, or cloxacillin; extended-spectrum beta-lactamase-producing (ESBL+) E coli or Klebsiella spp
were defined as E coli or Klebsiella spp isolates with
positive ESBL test results; carbapenem-resistant
Enterobacteriaceae (CRE) were defined as E coli
or Klebsiella spp with resistance to a carbapenem
(ertapenem, imipenem, or meropenem); multidrug-resistant
P aeruginosa (MRPA) demonstrated
simultaneous resistance to 11 drugs under four
classes of antibiotics (beta-lactams, carbapenems,
aminoglycosides, and fluoroquinolones); multidrug-resistant
Acinetobacter spp (MDRA) demonstrated
simultaneous resistance to 12 drugs under five classes
of antibiotics (cephalosporins, fluoroquinolones,
aminoglycosides, beta-lactams [± beta-lactamase
inhibitor], and carbapenems). Tests to identify MRPA
and MDRA were performed in accordance with
Hospital Authority guidelines, although piperacillin
assessment was omitted. Multidrug-resistant strains
of S pneumoniae have not been defined.
Data collection
The following data (concerning the previous calendar
year) were annually collected from the Infection
Control Teams of individual private hospitals:
identification number and date for admission or
attendance; location of specimen collection (in- or out-patient); specimen type (eg, sputum or mid-stream
urine) and specimen date (collection, request,
or laboratory registration); identification number of
isolates within the same specimen; and AST results of
each targeted bacterium. Only isolates from clinical
specimens (rather than screening specimens) were
submitted.
Antibiotic susceptibility testing results
The AST results were divided into three categories:
“susceptible”, “intermediate”, and “resistant”.
“Intermediate” and “resistant” were collectively
regarded as “non-susceptible” (NS). Interpretations
by private hospital microbiology laboratories were
based on Clinical Laboratory Standards Institute
definitions.
Data analysis
Repeated isolates were de-duplicated for each
calendar year using the first isolate in each
admission, location, specimen group, and targeted
bacterium. Importantly, some isolates may not
have been tested for susceptibility to all antibiotics
listed. The NS percentages for each antibiotic were
calculated based on the proportion of isolates tested
for that antibiotic. The Cochran–Armitage trend test
was used for temporal trends. P values <0.05 were
considered statistically significant. All analyses were
performed using Stata 14.2 (Stata Corp, College
Station [TX], US).
Ethical approval and reporting standards
Patient consent was not obtained because aggregated
patient data were used without identifying
information.2 Ethics approval was obtained. This
manuscript adheres to the STROBE statement
checklist of cross-sectional studies for items to be
included.
Results
The total number of isolates per year, NS percentages,
and MDRO percentages for isolates from both
in- and out-patients were calculated for S aureus
(Table 1), E coli (Table 2), Klebsiella spp (Table 3),
P aeruginosa (Table 4), Acinetobacter spp (Table 5),
and S pneumoniae (Table 6). Key in-patient results
are highlighted below.
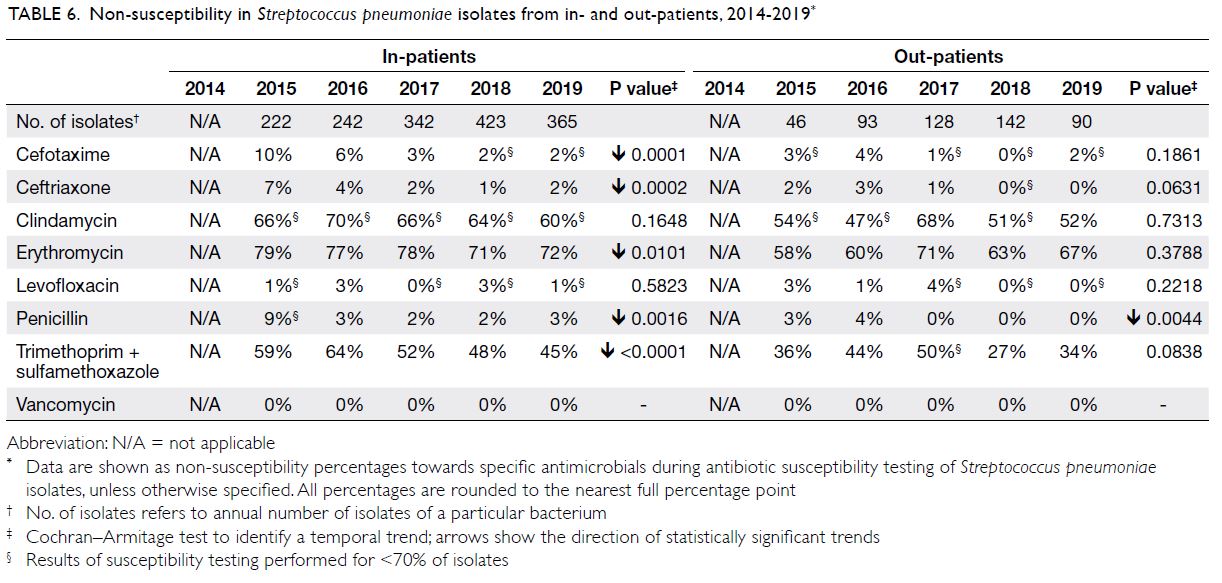
Table 6. Non-susceptibility in Streptococcus pneumoniae isolates from in- and out-patients, 2014-2019
Staphylococcus aureus
There were approximately 4100 to 5800 S aureus isolates per year (Table 1); respiratory specimens
comprised 50% and wound/pus swab specimens
comprised approximately 35% (online Supplementary
Table). The NS percentage for clindamycin ranged
from 24% to 31%. The NS percentages for co-trimoxazole
and fusidic acid were low (1%-2% and
3%-5%, respectively). Staphylococcus aureus showed
full susceptibility to both vancomycin and linezolid
(ie, NS percentages of 0%). The overall prevalence
of MRSA was 19% to 22%. For analysis of blood specimens, 29 to 73 isolates were obtained from
in-patients each year; of these, 10% to 18% were MRSA.
Escherichia coli
There were approximately 7900 to 9700 E coli isolates
per year (Table 2); nearly 70% were from urine and
approximately 10% were from wound/pus swabs.
The NS percentages for amoxicillin-clavulanate and
cefuroxime (parenteral) were moderately high (25%-33% and 36%-38%, respectively). The NS percentages
for fluoroquinolones were also moderately high (eg,
31%-37% for levofloxacin). The NS percentages for
nitrofurantoin and carbapenems were low (4%-8%
and 0%-1%, respectively). In terms of MDROs,
ESBL+ E coli demonstrated moderate prevalence
(25%-28%), while carbapenem-resistant E coli was
uncommon (0.1%-0.7%) among all isolates.
Klebsiella spp
There were approximately 2400 to 3400 Klebsiella isolates per year (Table 3); >30% were from urine
and >30% were from respiratory specimens. The NS
percentages were somewhat high: 16% to 24% for
amoxicillin-clavulanate, 25% to 30% for cefuroxime
(parenteral), 12% to 18% for levofloxacin, and 18%
to 26% for ciprofloxacin. The NS percentage for
carbapenems ranged from 0% to 2%, with an increasing
trend during the study period. In terms of MDROs,
ESBL+ Klebsiella demonstrated low prevalence (13%-17%), while carbapenem-resistant Klebsiella was
uncommon (0.2%-1.3%) among all isolates.
Pseudomonas aeruginosa
There were approximately 1300 to 1800 P aeruginosa isolates per year (Table 4); approximately 60%
were from respiratory specimens and 15% were from wound/pus swabs. The NS percentage for
the antipseudomonal beta-lactams piperacillin-tazobactam
was generally low (6%-11%), whereas it
was very high for ticarcillin-clavulanate (63%-74%).
The NS percentages for aminoglycosides were also
generally low (3%-11% for gentamicin and 1%-5%
for amikacin). The NS percentage for ciprofloxacin
remained consistent throughout the study period
(14%-15%). The prevalence of MRPA was very low
(0.0%-0.3%).
Acinetobacter spp
There were approximately 400 to 500 Acinetobacter
isolates per year (Table 5); they were mostly from
respiratory specimens, wound/pus swabs, and
urine (70%, 12%, and 10%, respectively). The NS
percentages for sulbactam-containing antibiotics
were 7% to 17% (ampicillin-sulbactam) and 8% to 15% (cefoperazone-sulbactam). The NS percentages
for fluoroquinolones (eg, ciprofloxacin) ranged from
13% to 25%. The NS percentages for carbapenems
were somewhat high values (8%-20% for imipenem
and 8%-19% for meropenem). The overall prevalence
of MDRA ranged from 2.2% to 7.8%.
Streptococcus pneumoniae
There were approximately 300 to 600 S pneumoniae isolates per year (Table 6); approximately 90% were
from respiratory specimens. The NS percentages for
beta-lactams were low (2%-9% for penicillin, 2%-10%
for cefotaxime, and 1%-7% for ceftriaxone). The NS
percentages for fluoroquinolones (eg, levofloxacin)
were low (0%-3%); the NS percentages for macrolides
(eg, erythromycin) were very high (71%-79%).
Streptococcus pneumoniae showed full susceptibility
to vancomycin (ie, NS percentage of 0%).
Discussion
To our knowledge, this is the first analysis of
susceptibility data among private hospitals in Hong
Kong. Such information provides important guidance
for clinical management and infection control
measures in the private sector. Here, we consider our
findings within local and international contexts.
Staphylococcus aureus
Staphylococcus aureus infections are usually treated
by amoxicillin-clavulanate, cloxacillin, or cefazolin
unless contra-indicated (eg, in cases of drug allergy)
or MRSA is suspected. For mild and superficial
infections, oral agents such as clindamycin and co-trimoxazole
can be considered, particularly when
such treatment is supported by AST results. Routine
combination treatment with aminoglycosides
for serious infections is no longer recommended
because this carries a risk of nephrotoxicity.7
Methicillin-resistant S aureus bacteraemia
is a serious condition with substantial mortality
(>30%).8 Methicillin-resistant S aureus is prevalent
in Hong Kong; in 2020, it comprised 43.1% of
S aureus isolates among all clinical specimens in
public hospitals, as well as 46.6% of isolates from blood cultures.9 Residential Care Home for the
Elderly (RCHE) resident carriage rates reportedly
range from 30.1% to 37.9%.10 11 In Australia, MRSA
is present in 17% to 22% of blood and other
specimens.12 In the UK, MRSA was present in 6.0%
of invasive isolates in 201913; this low rate could be
related to the extensive surveillance and infection
control efforts that resulted in a remarkable 86%
decrease in bloodstream infections (from 7700 to
1114 per year) from 2003 to 2012.14 Moreover, the
prevalence of MRSA among S aureus isolates from
human specimens decreased from 14% in 2013 to 7%
in 2017.15 The prevalence of methicillin resistance
should be considered when selecting empirical
therapy for patients with S aureus infections.
Vancomycin is a key component of therapy for
serious MRSA infections. Consistent with the low
prevalence of vancomycin resistance worldwide,16
vancomycin-resistant S aureus was absent from our
dataset. Staphylococcus aureus rarely demonstrates
resistance to linezolid17; as expected, S aureus
isolates in this study showed full susceptibility to
linezolid. However, although the NS percentages
for co-trimoxazole and fusidic acid were low, these
agents should serve as adjuncts only instead of
monotherapy in serious infections.
Escherichia coli and Klebsiella spp
Non-extended-spectrum beta-lactamaseproducing
isolates
Susceptible strains of E coli and Klebsiella spp are
usually treatable by amoxicillin-clavulanate or
cefuroxime.18 However, ESBL-producing strains
should be suspected in cases of serious infection
because of Enterobacteriaceae prevalence in Hong
Kong, where ESBL-producing E coli is regarded as a
critical MDRO.18
Extended-spectrum beta-lactamase-producing
isolates
Community spread is an important source of ESBL-related
infections; food animals are presumed
to serve as a major reservoir.19 For instance, the
isolation rate from pig offal is 52.4%.20 Among
public hospitals, the percentage of resistance to
third-generation cephalosporins (“3GC”) as a
surrogate marker for ESBL production among E coli
is approximately 26%9; this value is similar to our
findings. Furthermore, 17.0% to 18.6% of E coli
isolates from community urinary specimens
demonstrate ESBL-producing activity.9 Among RCHE residents, 55.9% were reported to be carriers
of ESBL-producing bacteria.9 In the UK, 12% of E coli isolates from blood specimens showed ESBL-producing activity15; Singaporean public hospitals identified ESBL-producing activity in 25.2% of
E coli isolates and 28.2% of Klebsiella isolates in
2017.21 From 2014 to 2019, the percentages of ESBL-producing isolates among Klebsiella isolates at
public hospitals in Hong Kong were 19% to 22%.9
Surveillance data regarding ESBL prevalence
can be affected by changes in laboratory practice
over time. Specifically, the Clinical and Laboratory
Standards Institute revised the cephalosporin
breakpoints in 2014, thus eliminating the need to
perform ESBL testing for clinical management—testing remains necessary for some infection control purposes and epidemiological investigations.22 However, not all laboratories have adopted the revised approach and the change remains controversial.23
The clinical specimen data in this study indicate that
all participating private hospitals have continued
to perform ESBL testing for Enterobacteriaceae
isolates.
For serious infections caused by ESBL-producing organisms, carbapenems are the most effective treatments.18
Carbapenem resistance
Our findings indicate that carbapenem resistance
remains uncommon but is increasing. Among
the known carbapenem resistance mechanisms,
carbapenemase production is the most important
from an infection control perspective, considering its propensity to spread to other organisms.24
Carbapenem-resistant Enterobacteriaceae is
often resistant to multiple classes of antibiotics,
which hinders treatment.25 The prevalence of this high-priority MDRO is increasing worldwide,5 6 presumably in relation to heightened awareness,
modified screening practices, and increased
transmission.26
Public hospitals in Hong Kong reported
increasing NS to carbapenem among E coli isolates
from 0.2% in 2016 to 0.4% in 2020 (NS percentage of 1.1%-1.8% for Klebsiella).9 Carbapenem-resistant E coli has become a major target of infection control
efforts in public hospitals.27 In contrast, CRE was not found among RCHE residents in a 2018 study.10
The limited treatment choices for CRE
infection include beta-lactam agents such as
ceftazidime-avibactam (inactive against metallo-beta-lactamases), aztreonam (active against metallo-beta-lactamases alone), and cefiderocol (active against all major classes of beta-lactamases); the
choices also include non-beta-lactam agents such as
intravenous colistin or tigecycline (if no alternative
is available). A single dose of oral fosfomycin may
be used for uncomplicated cystitis. Generally, these
agents are either less readily available in Hong Kong
(beta-lactams)28 or may cause severe adverse effects
(eg, nephrotoxicity for colistin and increased all-cause
mortality for tigecycline).29
Pseudomonas aeruginosa
Piperacillin ± tazobactam and ticarcillin-clavulanate are commonly recommended for the treatment
of P aeruginosa infections. Our data indicated
susceptibility to piperacillin-tazobactam and a lack
of susceptibility to ticarcillin-clavulanate. Thus, the
use of ticarcillin-clavulanate should be supported
by AST results. For serious infections, combination
treatment (eg, beta-lactam and aminoglycoside) may
be required to achieve synergistic effects.18
The prevalence of MRPA in our study was
consistently low (0.0%-0.3%), consistent with data
from public hospitals (0.02%-0.06% for 2014-2018).27 Data from other sources indicate higher prevalences
of MRPA (eg, 12%-14% among blood isolates,
according to the European Centre for Disease
Prevention and Control).30 However, the definition of MRPA can vary among sources.31 For instance, the
European Centre for Disease Prevention and Control
uses combined resistance to three or more antibiotic
groups. The strict definition of simultaneous
resistance to four antibiotic classes used in Hong
Kong may at least partially contribute to the overall
low prevalence.
Acinetobacter spp
Acinetobacter can survive for prolonged periods in dry environments, which facilitates nosocomial transmission.32 Sulbactam-containing beta lactams
are highly effective against Acinetobacter.18
Similar to MRPA, definitions of MDRA vary.
In the UK, multi-resistant Acinetobacter spp or
multi-resistant Acinetobacter baumannii (MRAB)
demonstrate co-resistance to aminoglycosides
and 3GC; the term MRAB-C refers to MRAB with
carbapenem resistance.33 Using an MDRA definition identical to ours, public hospitals reported a
decreasing MDRA prevalence (from 24% to 9% in
2014 to 201827); another study indicated that 0.6% of 1028 RCHE residents were carriers of MDRA.10
In analyses of carbapenem-resistant Acinetobacter
alone, the prevalence in public hospitals ranged
from 44% in 2014 to 53% in 201911; 9.1% of RCHE residents were carriers.11
Antibiotic-resistant Acinetobacter is classified
as a ‘critical threat’ by the World Health Organization
and an ‘urgent threat’ by the US Centers for
Disease Control and Prevention. Thus, although its
prevalence is decreasing, MDRA should be closely
monitored for any rebound.
Streptococcus pneumoniae
The primary treatments for invasive pneumococcal
infection are beta-lactams (penicillin G or 3GC) for
susceptible strains and vancomycin for penicillin-resistant
strains (plus 3GC for meningitis).
In Europe, the prevalence of penicillin
resistance among S pneumoniae isolates is
approximately 12% to 14% (2015-2019, invasive
isolates)30; the prevalence of macrolide resistance is approximately 14% to 16%. In Australia, these values are 3% to 6% and >20% to 25%, respectively.12 Our findings indicated a low NS percentage for penicillin but a very high NS percentage for macrolides; these
findings are compatible with the recommendation
that macrolides should not be used as monotherapy
during empirical treatment of infections in Hong
Kong.18 Fluoroquinolone resistance was previously reported to be high34 (>13.3% for levofloxacin),
although recent data from laboratory surveillance
by the CHP in the community setting indicate lower
resistance (0.0%-4.4% in 2014-2019).9 Our data are similar to the community values, as expected for an organism that most commonly causes community-acquired
pneumonia.18
Since the introduction of pneumococcal
vaccination, the disease burden caused by penicillin- and
erythromycin-resistant strains has decreased in
the US.5 In Hong Kong, approximately 180 invasive
pneumococcal infections are reported each year.35
Similar to other countries, Hong Kong has gradually
made pneumococcal vaccination available to
children, older adults, and high-risk individuals for
>10 years.35 As vaccine coverage increases, it would
be prudent to assess the changes in disease burden
caused by resistant strains of pneumococcus.
Implications
Compared with public hospitals, private hospitals
tend to have lower MDRO prevalences, particularly
for MRSA and MDRA, while following an overall
similar prevalence pattern (ie, increasing CRE,
stable ESBL, decreasing MDRA, and negligible
MRPA). Nonetheless, further MDRO monitoring
(particularly for CRE and MDRA) is warranted.
There may be multiple reasons for the
lower overall NS percentages, which could not
be assessed using the data collected in this study
(eg, case composition, antibiotic consumption,
and diagnostic practices). However, the physical
environment and isolation policy within private
hospitals may contribute to a generally lower
NS percentage. A key private hospital prescribes
single-room isolation for all MDRO carriers with
strict contact precautions.36 A more spacious
environment with fewer beds per cubicle could
theoretically lead to a lower cross-contamination
rate through indirect contact (eg, by shared toilets),
which is a main route for MDRO spread. With
respect to staffing, the infection-control-nurse-to-bed ratio may be more likely to meet (personal
communication) the level recommended by the CHP
(1:150 for acute hospitals).37 Sufficient single-patient
rooms and staffing (eg, nursing) are regarded as
crucial components of efforts to reduce healthcare-associated
colonisations and infections.38 39
Notably, the NS percentage was generally lower
among out-patient isolates than among in-patient
isolates, consistent with the reported literature.40
Strengths
First, the AST data were stratified by both location (in- and out-patient) and specimen groups. The
stratification of antibiogram data can facilitate
antibiotic stewardship programmes by exposing
important differences in susceptibility.41 Second,
the collected data spanned a 6-year period with a
large number of isolates, enabling the application
of a consistent methodology that can enhance trend
analysis accuracy. Third, MDRO prevalences were
collected; such data are not required by the World
Health Organization42 but are frequently regarded
as key information in international surveillance
reports.5 12 15 30
Limitations
Cautious interpretation of the findings is necessary. First, a subset of the antibiotic-bacterium
combinations were tested in a smaller proportion
of isolates (<70%), which could have led to biased
assessment. Second, because member hospital
laboratories had different levels and types of
accreditation, inter-laboratory practice variations
could have influenced the AST results. Third, the specimen group classification was arbitrary. Fourth,
differences in case composition among hospitals
may lead to misleading conclusions if direct head-to-head comparison is performed. Finally, CRE was
defined by susceptibility results, rather than specific
tests for carbapenemase detection.
Conclusion
Our findings provide important insights concerning antibiotic resistance at private hospitals in Hong
Kong. Although the overall situation in private
hospitals is considered satisfactory, there remains a
need for sustained efforts in resistance surveillance,
infection control, and antibiotic stewardship.
Author contributions
Concept or design: L Lui.
Acquisition of data: L Lui
Analysis or interpretation of data: All authors.
Drafting of the manuscript: L Lui.
Critical revision of the manuscript for important intellectual content: All authors.
Acquisition of data: L Lui
Analysis or interpretation of data: All authors.
Drafting of the manuscript: L Lui.
Critical revision of the manuscript for important intellectual content: All authors.
All authors had full access to the data, contributed to the
study, approved the final version for publication, and take
responsibility for its accuracy and integrity.
Conflicts of interest
All authors have disclosed no conflicts of interest.
Acknowledgement
Infection Control Branch would like to express her appreciation to private hospitals for providing the AST data
consistently over the years.
Membership of The Working Group of Collaboration between
CHP and Private Hospitals on Safe Use of Antibiotics and
Infection Control (in alphabetical order):
H Chen (Chairperson), Infection Control Branch, Centre for Health Protection, Department of Health, Hong Kong
Raymond WH Yung (Co-Chairperson), Hong Kong
Sanatorium & Hospital, Hong Kong
Ada Chan, Union Hospital, Hong Kong
WC Chan, Hong Kong Sanatorium & Hospital, Hong Kong
YM Cheng, Precious Blood Hospital (Caritas), Hong Kong
T Cheuk, Matilda International Hospital, Hong Kong
Christina Cheung, St Paul’s Hospital, Hong Kong
Eddie Cheung, Hong Kong Adventist Hospital–Stubbs Road, Hong Kong
Gary Cheung, Matilda International Hospital, Hong Kong
Joe Cheung, Hong Kong Adventist Hospital–Stubbs Road, Hong Kong
Billy SH Chui, Evangel Hospital, Hong Kong
August Fok, Hong Kong Adventist Hospital–Tsuen Wan, Hong Kong
Clara DK Kwok, Gleneagles Hospital Hong Kong, Hong Kong
Maggie MK Kwok, St Teresa’s Hospital, Hong Kong
Mooris Lai, Union Hospital, Hong Kong
Conita Lam, St Paul’s Hospital, Hong Kong
Wendy Lam, Canossa Hospital (Caritas), Hong Kong
MY Lau, Precious Blood Hospital (Caritas), Hong Kong
Patrick PL Lau, Hong Kong Baptist Hospital, Hong Kong
Andy Leung, Hong Kong Adventist Hospital–Tsuen Wan, Hong Kong
SL Loke, St Teresa’s Hospital, Hong Kong
L Lui, Infection Control Branch, Centre for Health Protection, Department of Health, Hong Kong
WH Seto, Gleneagles Hospital Hong Kong, Hong Kong
Winnie LH Wan, Evangel Hospital, Hong Kong
Cindy YY Wong, Hong Kong Baptist Hospital, Hong Kong
LC Wong, Infection Control Branch, Centre for Health Protection, Department of Health, Hong Kong
WO Wong, Canossa Hospital (Caritas), Hong Kong
KL Yan, Union Hospital, Hong Kong
PW Yu, Hong Kong Sanatorium & Hospital, Hong Kong
ST Yuen, St Paul’s Hospital, Hong Kong
H Chen (Chairperson), Infection Control Branch, Centre for Health Protection, Department of Health, Hong Kong
Raymond WH Yung (Co-Chairperson), Hong Kong
Sanatorium & Hospital, Hong Kong
Ada Chan, Union Hospital, Hong Kong
WC Chan, Hong Kong Sanatorium & Hospital, Hong Kong
YM Cheng, Precious Blood Hospital (Caritas), Hong Kong
T Cheuk, Matilda International Hospital, Hong Kong
Christina Cheung, St Paul’s Hospital, Hong Kong
Eddie Cheung, Hong Kong Adventist Hospital–Stubbs Road, Hong Kong
Gary Cheung, Matilda International Hospital, Hong Kong
Joe Cheung, Hong Kong Adventist Hospital–Stubbs Road, Hong Kong
Billy SH Chui, Evangel Hospital, Hong Kong
August Fok, Hong Kong Adventist Hospital–Tsuen Wan, Hong Kong
Clara DK Kwok, Gleneagles Hospital Hong Kong, Hong Kong
Maggie MK Kwok, St Teresa’s Hospital, Hong Kong
Mooris Lai, Union Hospital, Hong Kong
Conita Lam, St Paul’s Hospital, Hong Kong
Wendy Lam, Canossa Hospital (Caritas), Hong Kong
MY Lau, Precious Blood Hospital (Caritas), Hong Kong
Patrick PL Lau, Hong Kong Baptist Hospital, Hong Kong
Andy Leung, Hong Kong Adventist Hospital–Tsuen Wan, Hong Kong
SL Loke, St Teresa’s Hospital, Hong Kong
L Lui, Infection Control Branch, Centre for Health Protection, Department of Health, Hong Kong
WH Seto, Gleneagles Hospital Hong Kong, Hong Kong
Winnie LH Wan, Evangel Hospital, Hong Kong
Cindy YY Wong, Hong Kong Baptist Hospital, Hong Kong
LC Wong, Infection Control Branch, Centre for Health Protection, Department of Health, Hong Kong
WO Wong, Canossa Hospital (Caritas), Hong Kong
KL Yan, Union Hospital, Hong Kong
PW Yu, Hong Kong Sanatorium & Hospital, Hong Kong
ST Yuen, St Paul’s Hospital, Hong Kong
Funding/support
This research received no specific grant from any funding agency in the public, commercial, or not-for-profit sectors.
Ethics approval
This study was approved by the Hong Kong Department
of Health Ethics Review Board (Ref: LM 275/2021). The
requirement for patient consent was waived by the Ethics
Review Board.
References
1. Truong WR, Hidayat L, Bolaris MA, Nguyen L, Yamaki J.
The antibiogram: key considerations for its development
and utilization. JAC Antimicrob Resist 2021;3:dlab060. Crossref
2. Centre for Health Protection, Department of Health, Hong
Kong SAR Government. Antibiogram for six selected
bacterial isolates from private hospitals by in- and outpatient
setting for year 2014-2019. Available from: https://www.chp.gov.hk/files/pdf/antibiotic_sensitivity_table_private_hospitals.pdf. Accessed 21 Jul 2021.
3. Hong Kong SAR Government. Chapter 9 Health. Hong Kong Yearbook, 2019. Available from: https://www.yearbook.gov.hk/2019/en/pdf/E09.pdf. Accessed 6 Nov
2020.
4. Kong X, Yang Y, Gao J, et al. Overview of the health care
system in Hong Kong and its referential significance to
mainland China. J Chin Med Assoc 2015;78:569-73. Crossref
5. Centers for Disease Control and Prevention, US
Department of Health and Human Services. Antibiotic
resistance threats in the United States, 2019. Available
from: https://www.cdc.gov/drugresistance/pdf/threats-report/2019-ar-threats-report-508.pdf. Accessed 6 Nov 2020.
6. World Health Organization. Global priority list of
antibiotic-resistant bacteria to guide research, discovery,
and development of new antibiotics. Available from:
https://www.who.int/medicines/publications/WHO-PPL-Short_Summary_25Feb-ET_NM_WHO.pdf. Accessed 21 Jul 2021.
7. Lowy FD. Methicillin-resistant Staphylococcus aureus
(MRSA) in adults: treatment of bacteremia. UpToDate.
Available from: https://www.uptodate.com/contents/methicillin-resistant-staphylococcus-aureus-mrsa-in-adults-treatment-of-bacteremia. Accessed 5 Jul 2021.
8. You JH, Choi KW, Wong TY, et al. Disease burden, characteristics, and outcomes of methicillin-resistant
Staphylococcus aureus bloodstream infection in Hong
Kong. Asia Pac J Public Health 2017;29:451-61.Crossref
9. Centre for Health Protection, Department of Health,
Hong Kong SAR Government. Antimicrobial resistance
surveillance. Available from: https://www.chp.gov.hk/en/static/101603.html. Accessed 14 Apr 2021.
10. Chen H, Au KM, Hsu KE, et al. Multidrug-resistant organism carriage among residents from residential
care homes for the elderly in Hong Kong: a prevalence
survey with stratified cluster sampling. Hong Kong Med J
2018;24:350-60. Crossref
11. Cheng VC, Chen H, Wong SC, et al. Role of hand hygiene
ambassador and implementation of directly observed hand
hygiene among residents in residential care homes for
the elderly in Hong Kong. Infect Control Hosp Epidemiol
2018;39:571-7. Crossref
12. Australian Commission on Safety and Quality in Health
Care. AURA 2019: third Australian report on antimicrobial
use and resistance in human health. Available from: https://
www.safetyandquality.gov.au/our-work/antimicrobial-resistance/
antimicrobial-use-and-resistance-australia-surveillance-
system/aura-2019. Accessed 14 Apr 2021.
13. European Centre for Disease Prevention and Control.
Country summaries—antimicrobial resistance in the EU/
EEA 2019. Available from: https://www.ecdc.europa.eu/sites/default/files/documents/Country%20summaries-AER-EARS-Net%20202019.pdf. Accessed 28 Jul 2021.
14. Duerden B, Fry C, Johnson AP, Wilcox MH. The control
of methicillin-resistant Staphylococcus aureus blood
stream infections in England. Open Forum Infect Dis
2015;2:ofv035. Crossref
15. Veterinary Medicines Directorate, HM Government. UK one health report—Joint report on antibiotic use and
antibiotic resistance, 2013-2017. Available from: https://assets.publishing.service.gov.uk/government/uploads/system/uploads/attachment_data/file/921039/Ted_Final_version__1318703-v45-One_Health_Report_2019_FINAL-accessible.pdf. Accessed 14 Apr 2021.
16. Cong Y, Yang S, Rao X. Vancomycin resistant Staphylococcus
aureus infections: a review of case updating and clinical
features. J Adv Res 2019;21:169-76. Crossref
17. S Shariati A, Dadashi M, Chegini Z, et al. The global
prevalence of daptomycin, tigecycline, quinupristin/
dalfopristin, and Linezolid-resistant Staphylococcus aureus
and coagulase–negative staphylococci strains: a systematic
review and meta-analysis. Antimicrob Resist Infect
Control 2020;9:56. Crossref
18. Centre for Health Protection, Department of Health, Hong
Kong SAR Government. Ho PL, Wu TC, editors. Reducing
bacterial resistance with IMPACT—Interhospital Multi-disciplinary
Programme on Antimicrobial ChemoTherapy.
5th edition. 2017. Available from: http://www.chp.gov.hk/files/pdf/reducing_bacterial_resistance_with_impact.pdf.
Accessed 14 Apr 2021.
19. Ho PL, Chow KH, Lai EL, et al. Extensive dissemination
of CTX-M-producing Escherichia coli with multidrug
resistance to ‘critically important’ antibiotics among food
animals in Hong Kong, 2008-10. J Antimicrob Chemother
2011;66:765-8. Crossref
20. Sapugahawatte DN, Li C, Zhu C, et al. Prevalence
and characteristics of extended-spectrum-Β-lactamase-producing and carbapenemase-producing Enterobacteriaceae from freshwater fish and pork in wet markets of Hong Kong. mSphere 2020;5:e00107-20. Crossref
21. One Health Antimicrobial Resistance Working Group,
Ministry of Health, Singapore. One health report on
antimicrobial utilisation and resistance 2017. Available
from: https://www.moh.gov.sg/resources-statistics/reports/one-health-report-on-antimicrobial-utilisation-and-resistance-
2017. Accessed 5 Jul 2021.
22. Clinical and Laboratory Standards Institute. CLSI M100-
ED30:2020. Performance standards for antimicrobial
susceptibility testing, 30th ed. Available from: http://em100.edaptivedocs.net/GetDoc.aspx?doc=CLSI%20M100%20ED30:2020&scope=user. Accessed 6 Nov 2020.
23. Livermore DM, Andrews JM, Hawkey PM, et al. Are
susceptibility tests enough, or should laboratories still
seek ESBLs and carbapenemases directly? J Antimicrob
Chemother 2012;67:1569-77. Crossref
24. Centers for Disease Control and Prevention, US
Government. CRE technical information. Available from:
https://www.cdc.gov/hai/organisms/cre/technical-info.html#Transmitted. Accessed 14 Apr 2021.
25. Centers for Disease Control and Prevention, US
Government. Clinicians play a critical role in helping
to identify patients colonized or infected with CRE and
preventing its spread. Available from: https://www.cdc.gov/hai/organisms/cre/cre-clinicians.html. Accessed 14
Apr 2021.
26. Public Health Agency of Canada. Canadian antimicrobial
resistance surveillance system—Update 2018. Available
from: https://www.canada.ca/content/dam/phac-aspc/documents/services/publications/drugs-health-products/canadian-antimicrobial-resistance-surveillance-system-2018-report-executive-summary/pub1-eng.pdf. Accessed 14 Apr 2021.
27. Hospital Authority, Hong Kong SAR Government. Quality
and safety annual report 2018. Available from: https://www.ha.org.hk/haho/ho/psrm/EQnSReport2018.pdf.
Accessed 5 Jul 2021.
28. Drug Office, Department of Health, Hong Kong SAR
Government. Search Drug Database. Available from:
https://www.drugoffice.gov.hk/eps/do/en/consumer/search_drug_database.html. Accessed 28 Sep 2021.
29. United States Food and Drug Administration. FDA drug
safety communication: FDA warns of increased risk of
death with IV antibacterial Tygacil (tigecycline) and
approves new Boxed Warning. Available from: https://
www.fda.gov/drugs/drug-safety-and-availability/fda-drug-safety-communication-fda-warns-increased-risk-death-iv-antibacterial-tygacil-tigecycline. Accessed 28 Sep 2021.
30. European Centre for Disease Prevention and Control.
Antimicrobial resistance in the EU/EEA (EARS-Net)—annual epidemiological report for 2019. Available from: https://www.ecdc.europa.eu/sites/default/files/documents/surveillance-antimicrobial-resistance-Europe-2019.pdf. Accessed 28 Jul 2021.
31. Horcajada JP, Montero M, Oliver A, et al. Epidemiology
and treatment of multidrug-resistant and extensively
drug-resistant Pseudomonas aeruginosa infections. Clin
Microbiol Rev 2019;32:e00031-19.Crossref
32. Dijkshoorn L, Nemec A, Seifert H. An increasing threat in
hospitals: multidrug-resistant Acinetobacter baumannii.
Nat Rev Microbiol 2007;5:939-51.Crossref
33. Public Health England. Cookson B, Gergonne B, Barrett S, et al. Working party guidance on the control of multiresistant
Acinetobacter outbreaks. Public Health
England. 29 Aug 2006. Available from: https://www.gov.uk/government/publications/acinetobacter-working-party-guidance-on-the-control-of-multi-resistant-acinetobacter-outbreaks/working-party-guidance-on-the-control-of-multi-resistant-acinetobacter-outbreaks. Accessed 6 Nov 2020.
34. Ip M, Chau SS, Chi F, et al. Longitudinally tracking
fluoroquinolone resistance and its determinants in
penicillin-susceptible and -nonsusceptible Streptococcus
pneumoniae isolates in Hong Kong, 2000 to 2005.
Antimicrobial Agents Chemother 2007;51:2192-4. Crossref
35. Centre for Health Protection, Department of Health,
Hong Kong SAR Government. Invasive pneumococcal
disease. Available from: https://www.chp.gov.hk/en/features/32346.html. Accessed 14 Apr 2021.
36. Hong Kong Sanatorium & Hospital. Infection control.
Available from: https://www.hksh-hospital.com/en/patient-info/infection-control. Accessed 28 Jul 2021.
37. Scientific Committee on Infection Control, Centre for
Health Protection, Department of Health, Hong Kong SAR
Government. Recommendations on hospital infection
control system in Hong Kong. Available from: https://www.chp.gov.hk/files/pdf/recommendations_on_hospital_infection_control_system_in_hong_kong.pdf. Accessed 28
Jul 2021.
38. Weinstein RA, Stone PW, Pogorzelska M, Kunches L,
Hirschhorn LR. Hospital staffing and health care–associated infections: a systematic review of the literature.
Clin Infect Dis 2008;47:937-44.Crossref
39. Stiller A, Salm F, Bischoff P, Gastmeier P. Relationship
between hospital ward design and healthcare-associated
infection rates: a systematic review and meta-analysis.
Antimicrobial Resist Infect Control 2016;5:51. Crossref
40. Saperston KN, Shapiro DJ, Hersh AL, Copp HL. A
comparison of inpatient versus outpatient resistance
patterns of pediatric urinary tract infection. J Urol
2014;191:1608-13. Crossref
41. Barlam TF, Cosgrove SE, Abbo LM et al. Implementing
an antibiotic stewardship program: guidelines by the
Infectious Diseases Society of America and the Society
for Healthcare Epidemiology of America. Clin Infect Dis
2016;62:e51-77. Crossref
42. World Health Organization. Global Antimicrobial
Resistance and Use Surveillance System (GLASS). Available
from: https://www.who.int/initiatives/glass. Accessed 28
Jul 2021.