Hong Kong Med J 2016 Oct;22(5):410–9 | Epub 26 Aug 2016
DOI: 10.12809/hkmj154735
© Hong Kong Academy of Medicine. CC BY-NC-ND 4.0
ORIGINAL ARTICLE
Primary ventriculoperitoneal shunting outcomes: a multicentre clinical audit for shunt infection
and its risk factors
Working Group on Neurosurgical Outcomes Monitoring;
Peter YM Woo, MMedSc1;
HT Wong, FRCSEd (SN)1;
Jenny KS Pu, FRCSEd (SN)2;
WK Wong, FRCSEd (SN)3;
Larry YW Wong, FRCSEd (SN)4;
Michael WY Lee, FRCSEd (SN)5;
KY Yam, FRCSEd (SN)6;
WM Lui, FRCSEd (SN)2;
WS Poon, FRCSEd (SN)7
1 Department of Neurosurgery, Kwong Wah Hospital, Yaumatei, Hong Kong
2 Division of Neurosurgery, Department of Surgery, Queen Mary Hospital,
Pokfulam, Hong Kong
3 Department of Neurosurgery, Princess Margaret Hospital, Laichikok,
Hong Kong
4 Department of Neurosurgery, Queen Elizabeth Hospital, Jordan, Hong
Kong
5 Department of Neurosurgery, Pamela Youde Nethersole Eastern
Hospital, Chai Wan, Hong Kong
6 Department of Neurosurgery, Tuen Mun Hospital, Tuen Mun, Hong Kong
7 Division of Neurosurgery, Department of Surgery, Prince of Wales
Hospital, Shatin, Hong Kong
Corresponding author: Dr Peter YM Woo (wym307@ha.org.hk)
This paper was presented at the 21st Annual Scientific Meeting of the
Hong Kong Neurosurgical Society, 6 December 2014, Hong Kong.

Abstract
Objectives: To determine the frequency of primary
ventriculoperitoneal shunt infection among patients
treated at neurosurgical centres of the Hospital
Authority and to identify underlying risk factors.
Methods: This multicentre historical cohort study
included consecutive patients who underwent
primary ventriculoperitoneal shunting at a Hospital
Authority neurosurgery centre from 1 January
2009 to 31 December 2011. The primary endpoint
was shunt infection, defined as: (1) the presence
of cerebrospinal fluid or shunt hardware culture
that yielded the pathogenic micro-organism with
associated compatible symptoms and signs of central
nervous system infection or shunt malfunction; or
(2) surgical incision site infection requiring shunt
reinsertion (even in the absence of positive culture);
or (3) intraperitoneal pseudocyst formation (even in
the absence of positive culture). Secondary endpoints
were shunt malfunction, defined as unsatisfactory
cerebrospinal fluid drainage that required shunt
reinsertion, and 30-day mortality.
Results: A primary ventriculoperitoneal shunt was
inserted in 538 patients during the study period.
The mean age of patients was 48 years (range,
13-88 years) with a male-to-female ratio of 1:1.
Aneurysmal subarachnoid haemorrhage was the
most common aetiology (n=169, 31%) followed by
intracranial tumour (n=164, 30%), central nervous
system infection (n=42, 8%), and traumatic brain
injury (n=27, 5%). The mean operating time was 75
(standard deviation, 29) minutes. Shunt reinsertion
and infection rates were 16% (n=87) and 7%
(n=36), respectively. The most common cause for
shunt reinsertion was malfunction followed by
shunt infection. Independent predictors for shunt
infection were: traumatic brain injury (adjusted
odds ratio=6.2; 95% confidence interval, 2.3-16.8),
emergency shunting (2.3; 1.0-5.1), and prophylactic
vancomycin as the sole antibiotic (3.4; 1.1-11.0). The
30-day all-cause mortality was 6% and none were
directly procedure-related.
Conclusions: This is the first Hong Kong territory-wide
review of infection in primary ventriculoperitoneal
shunts. Although the ventriculoperitoneal
shunt infection rate met international standards,
there are areas of improvement such as vancomycin
administration and the avoidance of scheduling the
procedure as an emergency.
New knowledge added by this study
- The local rate of infection in ventriculoperitoneal (VP) shunts meets international standards.
- Vancomycin antibiotic prophylaxis is a risk factor for shunt infection and is a novel finding.
- VP shunt inserted as an emergency procedure is the strongest risk factor for infection.
- There is a need to review prophylactic vancomycin administration in terms of timing, dosage, and the need for its combination with another antibiotic.
- Emergency VP shunting is not recommended. Shunts should be implanted whenever possible as an elective procedure.
- A comprehensive local shunt surgery protocol to reduce the risk of shunt infection is recommended.
Introduction
Ventriculoperitoneal (VP) shunting is one of the
most common neurosurgical procedures performed
to treat patients with hydrocephalus, which is a
disorder related to an abnormal accumulation of
cerebrospinal fluid (CSF) in the brain. The operation
involves diverting CSF from the ventricles of the
brain to the peritoneal cavity of the abdomen
by catheter implantation. Despite being a well-established
procedure, shunt failure can be as high
as 70% in the first year with an annual occurrence
rate of 5% thereafter.1 One of the main causes for
failure is shunt infection, a potentially debilitating
complication that more than doubles the risk of
death and exposes affected patients to 3 times
as many neurosurgical procedures as non-infected
patients.2 Shunt infection varies and occurs in 3%
to 17% of patients. Standard management involves
intravenous antibiotic therapy, shunt removal,
insertion of an external ventricular drain, and
replacement with a new shunt once the patient’s
CSF is free of microbial infection.1 3 4 5 6 The economic
impact of VP shunt infection can be considerable.
In the US, the median cost per episode per patient
has been reported as US$23 500, accountable
for US$2.0 billion in annual hospital charges.7 8
Evidence suggests that the adoption of a strict
institutional implantation protocol can significantly
reduce the risk of this most challenging shunt
complications.9 10 11 12 This retrospective study aimed
to determine the frequency of primary VP shunt
reinsertions and infection among patients treated
in Hong Kong’s public health system and to identify
risk factors for shunt infection.
Methods
This was a multicentre retrospective study of patients
who underwent VP shunt implantation at all seven
Hong Kong Hospital Authority neurosurgical units.
The Hospital Authority is a public service highly
subsidised by the Hong Kong Special Administrative
Region Government, and responsible for delivering
health care for 90% of inpatient bed days in the city.13
Clinical research ethics committee approval was
obtained from the participating centres. Patients
who underwent primary VP shunting from 1
January 2009 to 31 December 2011 were included
in this study. Those who underwent alternative CSF
diversion procedures or those with a history
of VP shunt implantation were excluded from this
review. Data from clinical records, operation notes,
medication-dispensing records, CSF biochemistry,
cell counts, and microbiological cultures were
collected. The primary endpoint for this study was
primary VP shunt infection. The criteria for shunt
infection were: (1) CSF or shunt hardware culture
that yielded the pathogenic micro-organism with
associated compatible symptoms and signs of
central nervous system (CNS) infection or shunt
malfunction5 14 15; or (2) surgical incision site infection,
as defined by the National Nosocomial Infection
Surveillance System, requiring shunt reinsertion
(even in the absence of a positive culture)16; or (3)
intraperitoneal pseudocyst formation (even in the
absence of a positive culture). Secondary endpoints
were shunt malfunction, defined as unsatisfactory
CSF drainage that required shunt reinsertion, and
30-day mortality. Potential risk factors for shunt
infection were classified as patient-, disease-, or
surgical-related factors. All subjects were followed
up for at least 30 days from the operation date or
until death.
Statistical analysis was carried out using
Pearson’s Chi squared test, Fisher’s exact test, and
binary logistic regression to identify risk factors for
shunt infection. The Kaplan-Meier (log-rank) and
Cox proportional hazards models were employed
for survival analysis. Patient, disease, and surgical
factors were used as covariates and a stepwise
regression strategy was adopted (Table 1). P values of <0.05 were considered statistically significant. All
tests were performed using the Statistical Package
for the Social Sciences (Windows version 16.0.1;
SPSS Inc, Chicago [IL], US).
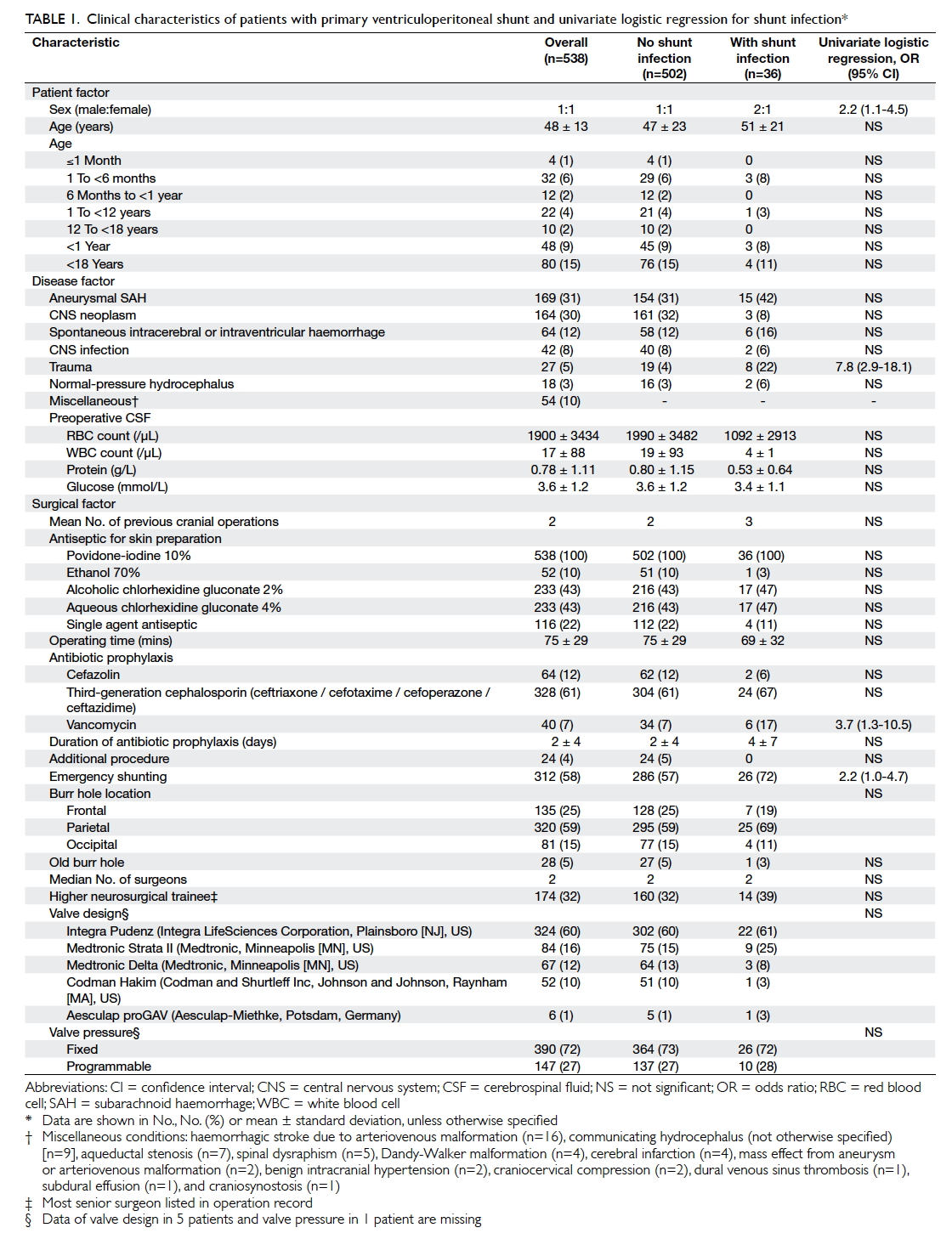
Table 1. Clinical characteristics of patients with primary ventriculoperitoneal shunt and univariate logistic regression for shunt infection
Results
During the 3-year period, 538 patients underwent
primary VP shunt implantation and 87% (n=470)
had complete clinical follow-up with a median
duration of 37 months (range, 3 days to 76 months).
Seven (1%) patients were transferred to other
hospitals within 30 days of the procedure. The
median duration of hospitalisation was 42 days
(range, 3 days to 36 months) and the median length
of time from admission to shunting was 18 days
(range, <1 day to 21 months). The clinical features
and surgical variables are presented in Table 1. The mean (± standard deviation) age of patients was 48
± 13 years (range, 13-88 years) and the male-to-female ratio was 1:1. In the study group, 80 (15%) were paediatric patients and 48 (9%) were infants. Overall, primary VP shunting
was performed for post-aneurysmal subarachnoid
haemorrhage communicating hydrocephalus in 169
(31%) patients, for CNS neoplasms in 164 (30%)
patients, and for spontaneous intracerebral or
intraventricular haemorrhage in 64 (12%) patients.
For patients who had preoperative CSF sampling
performed, the mean red blood cell count was 1900/µL, white cell count was 17/µL, total protein level
was 0.78 g/L, and glucose level was 3.6 mmol/L.
Over one quarter of patients (n=155, 29%)
had never had prior cranial neurosurgery and
approaching half had undergone either one (n=141,
26%) or two (n=115, 21%) previous procedures.
Antiseptic skin preparation was povidone-iodine
10% combined with another antiseptic in 422 (78%)
patients and with povidone iodine alone in the
remainder. The mean operating time for VP shunting
was 75 ± 29 minutes. All patients had antibiotic
prophylaxis of whom 328 (61%) were prescribed a third-generation cephalosporin and
40 (7%) had vancomycin. Twelve (2%)
patients had a rifampicin-clindamycin antibiotic-impregnated
ventricular catheter as part of the shunt
system. The majority of operations were performed
in an emergency setting (n=312, 58%) and shunt
implantation was the sole procedure performed
(n=514, 96%). The burr hole was most frequently
positioned at the parietal location in 320 (59%)
patients and 135 (25%) had a frontal burr hole. New
burr holes were fashioned for shunt placement 95%
of the time. The median number of surgeons was two,
with a third of shunts performed by higher neurosurgical
trainees (n=174, 32%) and the remaining performed
by a neurosurgical specialist. Almost three quarters
of VP shunts had a fixed-pressure valve (n=390,
72%) and the predominant design utilised was the
Integra Pudenz flushing valve (Integra LifeSciences
Corporation, Plainsboro [NJ], US) in 324 (60%)
patients.
The rate of VP shunt reinsertion was 16%
(n=87) and infection was 7% (n=36). The main
causes for reinsertion were malfunction (9%)
followed by infection. The annual proportion of
shunts that required reoperation or were infected
was comparable (P=0.87) [Fig 1]. The median time from shunt implantation to shunt removal for
infection was 64 days (range, 2 days to 10 months).
A cumulative risk for infection was noted affecting
3% of shunts in the first 30 days, 6% in 6 months,
and 7% in 1 year. Although 68 (13%) patients were
lost to follow-up, attrition analysis revealed that
this did not affect infection rates. The mean follow-up
duration in this subgroup between those with
infection and those without was comparable at 526
days and 554 days, respectively (P=0.43). In addition,
the incidence of shunt infection in patients with
incomplete follow-up (5%) was similar to those with
complete follow-up (7%) [P=0.42].
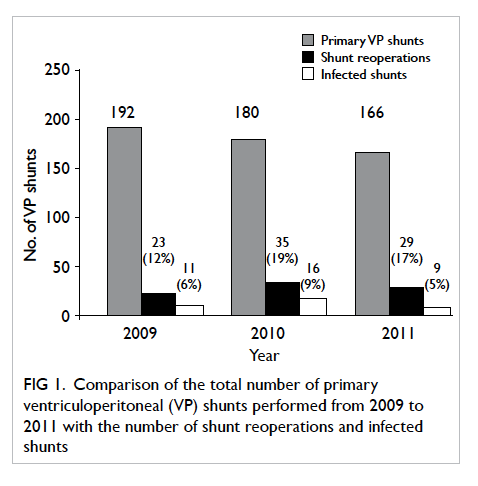
Figure 1. Comparison of the total number of primary ventriculoperitoneal (VP) shunts performed from 2009 to 2011 with the number of shunt reoperations and infected shunts
Most infections manifested as meningitis
or ventriculitis (n=19, 53%), followed by wound
breakdown (n=15, 42%) and peritonitis (n=2,
6%). The most common causative bacteria were
coagulase-negative staphylococci (CoNS) [n=25,
69%] of which methicillin resistance was detected
in 19 (76%) patients (Table 2). All CoNS species
were sensitive to vancomycin with a quarter of
methicillin-resistant (MR) species susceptible to
aminoglycosides such as gentamicin or amikacin.
The second most common infective agent affecting
four (11%) patients was MR Staphylococcus aureus
(MRSA). Polymicrobial infection was evident in
six (17%) patients. One patient with peritonitis had
mixed Gram-positive and -negative micro-organisms
from CSF cultures.
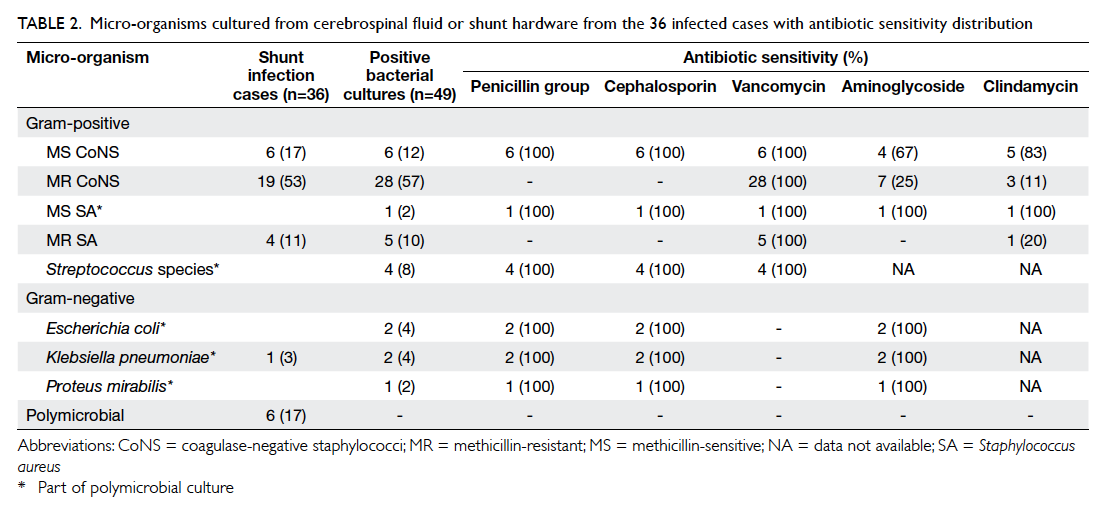
Table 2. micro-organisms cultured from cerebrospinal fluid or shunt hardware from the 36 infected cases with antibiotic sensitivity distribution
The only patient risk factor for shunt infection
was sex (Table 1). Male patients had a greater than two-fold increased odds of infection (odds ratio [OR]=2.2; 95% confidence interval [CI], 1.1-4.5). Traumatic brain injury (TBI) was the
only disease risk factor (OR=7.8; 95% CI, 2.9-18.1).
Surgical factors included the use of vancomycin
as the prophylactic antibiotic (OR=3.7; 95% CI,
1.3-10.5) and shunts implanted as an emergency
procedure (OR=2.2; 95% CI, 1.0-4.7). After adjusting
for confounding factors, the independent risk factors
for primary VP shunt infection were TBI (adjusted
OR=6.2; 95% CI, 2.3-16.8), the use of vancomycin
(adjusted OR=3.4; 95% CI, 1.1-11.0), and emergency
shunting (adjusted OR=2.3; 95% CI, 1.0-5.1) [Table 3].
With respect to shunt infection, there was
a difference in duration of shunt implantation for
patients with the aforementioned risk factors as
demonstrated by the significant separation of Kaplan-Meier survival curves (Fig 2). This was ascertained
by Cox regression analysis (Table 3). Median shunt
survival for trauma patients was 35 days (vs 154 days
in non-trauma patients), 32 days for patients who
received vancomycin (vs 124 days for alternative
antibiotics), and 65 days for emergency operations
(vs 208 days for elective operations).
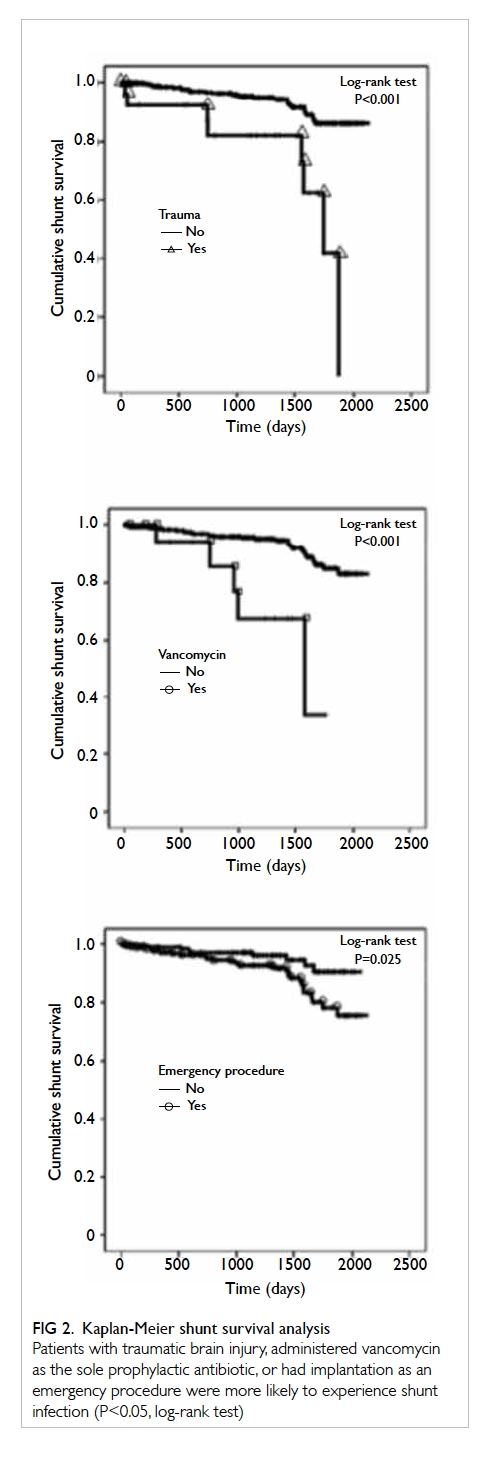
Figure 2. Kaplan-Meier shunt survival analysis
Patients with traumatic brain injury, administered vancomycin as the sole prophylactic antibiotic, or had implantation as an emergency procedure were more likely to experience shunt infection (P<0.05, log-rank test)
In this study, 30-day all-cause mortality
was 6% (n=32), but none was directly procedure-related.
Almost half of these patients (n=15, 47%)
had an underlying malignant CNS tumour; the
majority being brain metastases (n=12, 80%). After
accounting for patient age, sex, disease aetiology,
shunt reinsertion and infection, a diagnosis of
malignant brain tumour was the only significant
independent predictor for 30-day mortality with an
adjusted OR of 5.6 (95% CI, 2.6-11.7).
Discussion
Ventriculoperitoneal CSF shunting has considerably
reduced the morbidity and mortality of patients with
hydrocephalus since its first description in 1908.17
More than a century later the operation remains
the mainstay treatment for this condition. Despite
the introduction of antibiotics, improvements
in shunt materials and surgical techniques, VP
shunt complications are common. Long-term
epidemiological studies have indicated that more
than half of all patients with CSF shunts will
require a surgical revision in their lifetime.4 18 Shunt infection is a serious complication with potentially
devastating consequences. Observational studies
have recorded infection rates ranging between 3%
and 17%, but more consistent estimates from larger
patient cohorts cite rates of 6% to 8%.1 3 4 5 Our local
shunt infection rate of 7% is relatively lower than
other previously published findings and is in keeping
with the results from other developed countries.
The wide range of shunt infection rates
quoted in the literature is due in part to the diverse
definitions adopted for shunt infection and the
patient populations studied. Many studies have
defined infection as a positive CSF microbial culture
or the presence of CSF pleocytosis or low CSF glucose
levels with clinical features of CNS infection.3 11 19
Due to the study design, a more pragmatic definition
was adopted whereby infection was determined
retrospectively by either a positive CSF or shunt
hardware microbial culture in the presence of shunt
malfunction.5 14 15 Nonetheless, it is acknowledged
that the true incidence of shunt infection may be
overestimated by false-positive cultures from skin
flora. The reasons for selecting this interpretation
for shunt infection were three-fold. First, it allowed
for micro-organism identification and consequent
epidemiological analysis; second, infection may
not be clinically apparent with malfunctioning
shunts; and third, CSF cultures alone cannot exclude
infection in cases of shunt malfunction.15
The only disease risk factor independently
associated with VP shunt infection was TBI.
This may be due to two reasons. Delayed post-traumatic
hydrocephalus invariably occurs in
severe TBI patients and develops in over a third of
those subject to decompressive craniectomies.20
Such patients often have a prolonged hospital stay
and undergo multiple operations before a shunt is
eventually implanted. In this cohort, TBI patients
had a mean duration of hospitalisation of 89 days,
which was 2 weeks more than the mean stay of 74
days for hydrocephalic patients with alternative
neurosurgical conditions. Protracted hospitalisation
may lead to skin colonisation with drug-resistant
organisms that can evade single-agent conventional
antibiotic prophylaxis.21 This is supported by
evidence from this study where causative bacteria
of shunt infection were resistant to the prescribed
prophylactic antibiotic in 80% of TBI patients.
These patients also had a mean number of three
prior cranial procedures before shunting compared
with two operations in patients with non-traumatic
hydrocephalus aetiology. Previous surgery is well
known to be a main cause of CSF leak in shunted
patients and contributes to an increased risk of
infection.5 22 Although in this patient series, the
number of prior cranial procedures per se did not
impart greater risk, detailed clinical data regarding
CSF leak in TBI patients were not collected and
therefore the influence of TBI on infection can only
be inferred.
An unexpected finding was that patient age
was not a risk factor for shunt infection. This is in
contrast to several larger studies that identified
paediatric patients, especially infants (younger
than a year), to be particularly at risk.11 23 Infants have less-developed humoral and cellular immune
systems with immature skin growth rendering them
more vulnerable to shunt infection. A likely reason
for this observation is the small number of paediatric
patients (n=80, 15%) in this cohort with only 9%
(n=48) being infants. Larger sample size may
delineate more distinctive differences among age-groups.
There is little doubt that systemic antibiotic
prophylaxis can prevent shunt infection.24 We,
however, interestingly identified the sole use of
vancomycin as a risk factor for shunt infection, a novel
observation that has not been previously reported in
the literature. The antibiotic was regularly reserved
for patients allergic to penicillin-group antibiotics
or for those with documented penicillin-resistant
microbial infection or colonisation. This finding
apparently seems counter-intuitive especially when
all causative CoNS identified in this cohort were
sensitive to vancomycin. The issue may lie with the
timing of its administration before the procedure
and its dosage. With regard to timing, systemic
vancomycin requires slow intravenous infusion to
reduce the risk of a hypersensitivity reaction that
manifests as either red man syndrome or anaphylaxis
and occurs in 3.7% to 47% of patients.25 To further
illustrate the incidence of these symptoms, the first
randomised controlled trial investigating its efficacy
in shunt procedures was prematurely discontinued
due to these adverse effects.26 Most hospital
protocols require infusion rates over an hour as a
minimum, but clinical trials have demonstrated that
even lengthier 2-hour infusions can further reduce
the frequency and severity of these reactions.25 27 Furthermore, the efficacy of vancomycin to treat
CoNS and MRSA infections has been questioned
due to observations of slower bactericidal activity,
compared with nafcillin, than was previously
recognised.28 To address this issue we suggest that
rigid guidelines should be adhered to with respect
to the adequate timing of vancomycin infusion
before skin incision. Should more rapid infusions
be required, for example, in the emergency setting,
pre-administration of intravenous diphenhydramine
before vancomycin infusion can prevent the
development of red man syndrome.25
Limited data are available about the
pharmacokinetics and CSF concentrations of
vancomycin in neurosurgical patients. In a
study reviewing intra-operative serum and CSF
vancomycin concentrations of paediatric patients
undergoing shunt implantation, the authors noted
that CSF penetration was negligible in patients with
non-inflamed meninges despite presumed adequate
loading doses.29 This was echoed in a later study
determining that among non-meningitic patients,
vancomycin CNS penetration was poor with a
CSF-to-serum ratio of only 18%.30 Its increasing use over
the course of decades has also led to a corresponding
rise in minimum bactericidal concentrations of
CoNS.28 These unique findings should prompt an
extensive review of prophylactic vancomycin use as
there is currently no consensus on a recommended
loading dose for neurosurgical procedures. In the
meantime, researchers have attempted to improve
the bactericidal activity in patients treated with
vancomycin with varying measures of success.
Vancomycin in combination with gentamicin results
in more rapid bactericidal rates in animal models28
and has been proven to be as effective as third-generation
cephalosporins in preventing surgical
site infection for neurosurgical procedures in a
randomised trial.31 Others have proposed intra-operative combined vancomycin-aminoglycoside
administration either intraventricularly, for shunt
hardware antibiotic bath immersion prior to
implantation or applied in powder form within the
subgaleal space of the wound with tenable positive
results.11 32 33 34
Antibiotic-impregnated (by rifampicin with
either clindamycin or minocycline) and silver-coated
ventricular catheters offer the greatest
promise in preventing shunt infection.35 36 There
exists a growing body of evidence in support of
antibiotic-impregnated ventricular catheters and
they are gradually replacing conventional plain
silicone catheters in daily practice with considerable
cost savings.37 38 39 40 There are also accompanying
concerns about the development of antibiotic-resistant
micro-organisms and a recent meta-analysis
has elucidated the higher risk of Gram-negative
and MRSA shunt infections.38 In this series, only 12
patients received antibiotic-impregnated catheters
during the study period so it is difficult to draw any
conclusion about their effectiveness.
Emergency VP shunting is another surgical-related
risk factor for infection and was performed
in more than half of patients who underwent the
procedure. Its significance is the greatest among
the three independent factors identified and is
possibly the most amenable to change in current
practice. The clinical condition of most patients with
hydrocephalus who require primary VP shunting
does not warrant emergency surgery although a
few indications exist, for example, obstructive pineal
region or cerebellar tumours that may present with
acute symptoms. More than two thirds of patients
(70%) in this study had conditions that necessitated
delayed shunting when the primary disease had been
treated and the patients stabilised. It is most likely
because of limited availability of operating theatre
among other related resources and the general
practice that VP shunting is delegated to more junior
members of the surgical team that this phenomenon
prevails. Several reasons support why ‘emergency’
primary shunting should be discouraged. It has
long been established by several protocol-driven
trials that shunting should be performed as the first
procedure of the operative day to minimise the risk
of contamination.9 10 11 To illustrate, a surgical incision
time after 10 am was observed to be a predictor for
infection.5 In elective procedures the neurosurgeon
in-charge and other responsible operating theatre
staff are likely to be more experienced in comparison
with personnel involved in emergencies. In particular,
it has been shown that individual surgical experience
is an important factor for infection with researchers
stating a higher incidence among neurosurgical
trainees or in surgeons who performed fewer than
147 shunts within a decade.4 41 Nonetheless, using
the former stratification of trainee versus specialist,
this was not evident in our cohort. Another
argument against ‘emergency’ VP shunting could
be the location where the procedure is performed.
For a variety of resource allocation reasons, shunting
scheduled as an emergency procedure is often not
performed in neurosurgery-designated operating
theatres. A study investigating the distribution of
bacteria in the operating room environment and
its relationship with ventricular shunt infections
concluded that positive environmental cultures
were more likely to occur in a theatre not devoted
to neurosurgery.42 Although procedure timing and
location were not explored in this audit, it is believed
that they were the principal explanations why shunts
implanted as an emergency were more likely to
become infected.
The time interval from shunt implantation
to revision for infection in our study is longer than
most published data with a median shunt survival
of 64 days.5 34 Our data show that 92% (n=33) of
shunt infections occurred within 6 months and is
compatible with the commonly held belief that the
infection begins intra-operatively with the inoculation
of skin flora, either from the patient or surgeon, into
the surgical wound.42 43 44 This is further substantiated
by the predominance of CoNS and S aureus in
81% of bacterial cultures in this patient series and
similarly in several previous reports.4 5 9 10 11 12 32 35 36 43
Coupled with positive research findings that theatre
discipline during surgery reduces infection risk, it
seems reasonable to conclude that institutional shunt
implantation protocols should be established.9 10 11 12 32
Even though the performance of our
neurosurgical community with regard to primary
VP shunt infection meets international standards,
there is room for improvement. The implementation
of a standardised shunt surgery protocol that covers
preoperative preparation as well as intra-operative
and postoperative management has consistently been
proven to be effective in reducing infection.9 10 11 12 32 The
landmark study by Choux at al10 first demonstrated
that meticulous measures—such as adopting a no-touch
technique for shunt handling, limiting the
length of shunt exposure time and the number of
people in the operating room—have dramatically
decreased shunt infection rates from 16% to less than
1%. It is our belief that a similarly comprehensive
protocol should be developed and based on the
findings of this preliminary study.
This study has several limitations. Data
collection was retrospective so key clinical
information such as the presence of CSF leak and
patient co-morbidities were missing. This may have
led to inadequate control for confounding factors.
An additional limitation inherent in studies of this
nature is the potential presence of observational bias
where data were collected without blinding after
outcomes were known. Follow-up was incomplete
with 68 (13%) patients defaulting from clinical
review over the course of 3 years. Inadequate follow-up
duration was also noted; seven (1%) patients were
transferred to other hospitals within 30 days of the
procedure and this might have influenced 30-day
all-cause mortality findings. Finally, our definition
of shunt infection did not include abnormal CSF
biochemistry criteria that could have confirmed or
refuted positive culture results of specimens that
might have been contaminated during collection.
Conclusions
This is the first territory-wide review of infection in
primary VP shunts conducted in Hong Kong’s public
health care setting. This study is also one of the
largest in the literature examining shunt infection
complications among a predominantly Chinese
population. Shunt infection was the second most
common cause for reinsertion occurring in 7% of
patients. Significant independent predictors for
shunt infection were TBI, vancomycin administration
for prophylaxis, and procedures performed in an
emergency setting. Although VP shunt infection
rates meet international standards, there are areas of
improvement that can be readily addressed such as
the timing or dosage of vancomycin and the avoidance
of performing the procedure as an emergency. The
best approach to reducing shunt infection may be
the design and adoption of a standardised shunt
surgery protocol customised to local practice.
Acknowledgements
We would like to thank members of the Hospital
Authority Head Office (HAHO) Co-ordinating
Committee (Neurosurgery), the Clinical Effectiveness
& Technology Management Department, the Division of Quality and Safety,
and the Clinical Data Analysis and Reporting System Team, HAHO IT Service for their
administrative advice and data collection. We also
wish to thank Drs Chris YW Liu, Alphon HY Ip, and Claudia Law for
their contributions in data collection and entry. This study was supported by the Tung Wah Group of Hospitals Neuroscience Research Fund.
Declaration
All authors have disclosed no conflicts of interest.
References
1. Wong JM, Ziewacz JE, Ho AL, et al. Patterns in
neurosurgical adverse events: cerebrospinal fluid shunt
surgery. Neurosurg Focus 2012;33:E13. Crossref
2. Schoenbaum SC, Gardner P, Shillito J. Infections of
cerebrospinal fluid shunts: epidemiology, clinical
manifestations, and therapy. J Infect Dis 1975;131:543-52. Crossref
3. Birjandi A, Zare E, Hushmandi F. Ventriculoperitoneal
shunt infection: a review of treatment. Neurosurg Q
2012;22:145-8. Crossref
4. Borgbjerg BM, Gjerris F, Albeck MJ, Børgesen SE. Risk of
infection after cerebrospinal fluid shunt: an analysis of 884
first-time shunts. Acta Neurochir (Wien) 1995;136:1-7. Crossref
5. Korinek AM, Fulla-Oller L, Boch AL, Golmard JL, Hadiji
B, Puybasset L. Morbidity of ventricular cerebrospinal fluid
shunt surgery in adults: an 8-year study. Neurosurgery
2011;68:985-94; discussion 994-5. Crossref
6. Patwardhan RV, Nanda A. Implanted ventricular shunts
in the United States: the billion-dollar-a-year cost of
hydrocephalus treatment. Neurosurgery 2005;56:139-44;
discussion 144-5. Crossref
7. Simon TD, Riva-Cambrin J, Srivastava R, et al. Hospital
care for children with hydrocephalus in the United States:
utilization, charges, comorbidities, and deaths. J Neurosurg
Pediatr 2008;1:131-7. Crossref
8. Shannon CN, Simon TD, Reed GT, et al. The economic
impact of ventriculoperitoneal shunt failure. J Neurosurg
Pediatr 2011;8:593-9. Crossref
9. Faillace WJ. A no-touch technique protocol to diminish
cerebrospinal fluid shunt infection. Surg Neurol
1995;43:344-50. Crossref
10. Choux M, Genitori L, Lang D, Lena G. Shunt implantation:
reducing the incidence of shunt infection. J Neurosurg
1992;77:875-80. Crossref
11. Kestle JR, Riva-Cambrin J, Wellons JC 3rd, et al. A
standardized protocol to reduce cerebrospinal fluid shunt
infection: the Hydrocephalus Clinical Research Network
Quality Improvement Initiative. J Neurosurg Pediatr
2011;8:22-9. Crossref
12. Pirotte BJ, Lubansu A, Bruneau M, Loqa C, Van Cutsem
N, Brotchi J. Sterile surgical technique for shunt placement
reduces the shunt infection rate in children: preliminary
analysis of a prospective protocol in 115 consecutive
procedures. Childs Nerv Syst 2007;23:1251-61. Crossref
13. World Health Organization and Department of Health,
Hong Kong. Hong Kong (China) health service delivery
profile, 2012. Available from: http://www.wpro.who.int/health_services/service_delivery_profile_hong_kong_(china).pdf. Accessed Mar 2016.
14. Overturf GD. Defining bacterial meningitis and other
infections of the central nervous system. Pediatr Crit Care
Med 2005;6 Suppl:S14-8. Crossref
15. Vanaclocha V, Sáiz-Sapena N, Leiva J. Shunt malfunction
in relation to shunt infection. Acta Neurochir (Wien)
1996;138:829-34. Crossref
16. Horan TC, Gaynes RP, Martone WJ, Jarvis WR, Emori TG.
CDC definitions of nosocomial surgical site infections,
1992: a modification of CDC definitions of surgical wound
infections. Infect Control Hosp Epidemiol 1992;13:606-8. Crossref
17. Kausch W. Die behandlung des hydrocephalus der kleinen
kinder. Arch Klin Chir 1908;87:709-96.
18. Tuli S, Drake J, Lawless J, Wigg M, Lamberti-Pasculli
M. Risk factors for repeated cerebrospinal shunt failures
in pediatric patients with hydrocephalus. J Neurosurg
2000;92:31-8. Crossref
19. Odio C, McCracken GH Jr, Nelson JD. CSF shunt infections
in pediatrics. A seven-year experience. Am J Dis Child
1984;138:1103-8. Crossref
20. Honeybul S, Ho KM. Incidence and risk factors for
post-traumatic hydrocephalus following decompressive
craniectomy for intractable intracranial hypertension and
evacuation of mass lesions. J Neurotrauma 2012;29:1872-8. Crossref
21. Wang KW, Chang WN, Shih TY, et al. Infection of
cerebrospinal fluid shunts: causative pathogens, clinical
features, and outcomes. Jpn J Infect Dis 2004;57:44-8.
22. Jeelani NU, Kulkarni AV, Desilva P, Thompson DN,
Hayward RD. Postoperative cerebrospinal fluid wound
leakage as a predictor of shunt infection: a prospective
analysis of 205 cases. Clinical article. J Neurosurg Pediatr
2009;4:166-9. Crossref
23. Davis SE, Levy ML, McComb JG, Masri-Lavine L.
Does age or other factors influence the incidence of
ventriculoperitoneal shunt infections? Pediatr Neurosurg
1999;30:253-7. Crossref
24. Ratilal B, Costa J, Sampaio C. Antibiotic prophylaxis for
surgical introduction of intracranial ventricular shunts.
Cochrane Database Syst Rev 2006;(3):CD005365. Crossref
25. Sivagnanam S, Deleu D. Red man syndrome. Crit Care
2003;7:119-20. Crossref
26. Odio C, Mohs E, Sklar FH, Nelson JD, McCracken GH Jr.
Adverse reactions to vancomycin used as prophylaxis for
CSF shunt procedures. Am J Dis Child 1984;138:17-9. Crossref
27. Healy DP, Sahai JV, Fuller SH, Polk RE. Vancomycin-induced
histamine release and “red man syndrome”:
comparison of 1- and 2-hour infusions. Antimicrob Agents
Chemother 1990;34:550-4. Crossref
28. Stevens DL. The role of vancomycin in the treatment
paradigm. Clin Infect Dis 2006;42 Suppl 1:S51-7. Crossref
29. Fan-Havard P, Nahata MC, Bartkowski MH, Barson
WJ, Kosnik EJ. Pharmacokinetics and cerebrospinal
fluid (CSF) concentrations of vancomycin in pediatric
patients undergoing CSF shunt placement. Chemotherapy
1990;36:103-8. Crossref
30. Albanèse J, Léone M, Bruguerolle B, Ayem ML,
Lacarelle B, Martin C. Cerebrospinal fluid penetration
and pharmacokinetics of vancomycin administered by
continuous infusion to mechanically ventilated patients
in an intensive care unit. Antimicrob Agents Chemother
2000;44:1356-8. Crossref
31. Pons VG, Denlinger SL, Guglielmo BJ, et al. Ceftizoxime
versus vancomycin and gentamicin in neurosurgical
prophylaxis: a randomized, prospective, blinded clinical
study. Neurosurgery 1993;33:416-22; discussion 422-3. Crossref
32. Choksey MS, Malik IA. Zero tolerance to shunt infections:
can it be achieved? J Neurol Neurosurg Psychiatry
2004;75:87-91.
33. Abdullah KG, Attiah MA, Olsen AS, Richardson A, Lucas
TH. Reducing surgical site infections following craniotomy:
examination of the use of topical vancomycin. J Neurosurg
2015;123:1600-4. Crossref
34. Ragel BT, Browd SR, Schmidt RH. Surgical shunt infection:
significant reduction when using intraventricular and
systemic antibiotic agents. J Neurosurg 2006;105:242-7. Crossref
35. Keong NC, Bulters DO, Richards HK, et al. The SILVER
(Silver Impregnated Line Versus EVD Randomized trial): a
double-blind, prospective, randomized, controlled trial of
an intervention to reduce the rate of external ventricular
drain infection. Neurosurgery 2012;71:394-403; discussion
403-4. Crossref
36. Sciubba DM, Stuart RM, McGirt MJ, et al. Effect of
antibiotic-impregnated shunt catheters in decreasing
the incidence of shunt infection in the treatment of
hydrocephalus. J Neurosurg 2005;103 Suppl:131-6. Crossref
37. Thomas R, Lee S, Patole S, Rao S. Antibiotic-impregnated
catheters for the prevention of CSF shunt infections: a
systematic review and meta-analysis. Br J Neurosurg
2012;26:175-84. Crossref
38. Konstantelias AA, Vardakas KZ, Polyzos KA, Tansarli GS,
Falagas ME. Antimicrobial-impregnated and -coated shunt
catheters for prevention of infections in patients with
hydrocephalus: a systematic review and meta-analysis. J
Neurosurg 2015;122:1096-112. Crossref
39. Parker SL, Farber SH, Adogwa O, Rigamonti D, McGirt MJ.
Comparison of hospital cost and resource use associated
with antibiotic-impregnated versus standard shunt
catheters. Clin Neurosurg 2011;58:122-5. Crossref
40. Parker SL, McGirt MJ, Murphy JA, Megerian JT, Stout
M, Engelhart L. Cost savings associated with antibiotic-impregnated
shunt catheters in the treatment of adult and
pediatric hydrocephalus. World Neurosurg 2015;83:382-6. Crossref
41. Cochrane DD, Kestle JR. The influence of surgical operative
experience on the duration of first ventriculoperitoneal
shunt function and infection. Pediatr Neurosurg
2003;38:295-301. Crossref
42. Duhaime AC, Bonner K, McGowan KL, Schut L, Sutton
LN, Plotkin S. Distribution of bacteria in the operating
room environment and its relation to ventricular
shunt infections: a prospective study. Childs Nerv Syst
1991;7:211-4. Crossref
43. Bayston R, Lari J. A study of the sources of infection in
colonised shunts. Dev Med Child Neurol 1974;16 Suppl
32:16-22. Crossref
44. Tulipan N, Cleves MA. Effect of an intraoperative double-gloving
strategy on the incidence of cerebrospinal fluid
shunt infection. J Neurosurg 2006;104 Suppl:5-8. Crossref