Hong Kong Med J 2015 Feb;21(1):61–8 | Epub 16 Jan 2015
DOI: 10.12809/hkmj144367
© Hong Kong Academy of Medicine. CC BY-NC-ND 4.0
MEDICAL PRACTICE
Prevention of ventilator-associated pneumonia
Arthur CW Lau, FHKAM (Medicine)1;
HM So, FHKAN (Critical Care)1;
SL Tang, BSc (Nursing)2;
Alwin Yeung, FHKAM (Medicine)2;
SM Lam, FHKAM (Medicine)1;
WW Yan, FHKAM (Medicine)1;
for the Hong Kong East Cluster Task Force on Prevention of Ventilator-associated Pneumonia in Critical Care Areas
1Department of Intensive Care, Pamela Youde Nethersole Eastern
Hospital, Chai Wan, Hong Kong
2Cardiac and Intensive Care Unit, Ruttonjee Hospital, Wanchai, Hong Kong
Corresponding author: Dr Arthur CW Lau (laucw3@ha.org.hk)

Abstract
Ventilator-associated pneumonia is the commonest,
yet mostly preventable, infection in mechanically
ventilated patients. Successful control of ventilator-associated
pneumonia can save hospitalisation
cost, and is possible by using a multidisciplinary
clinical and administrative approach. The ventilator-associated
pneumonia rate should be expressed as
the number of ventilator-associated pneumonia
days per 1000 ventilator days to take into account
the device-utilisation duration for meaningful
comparison. Various strategies address the issue,
including general infection control measures, body
positioning, intubation and mechanical ventilation,
oral and gastro-intestinal tract, endotracheal tube,
airway pressure, cuff pressure, selective digestive
and/or oropharyngeal decontamination, and
probiotic or early antibiotic treatment, as well as
overall administration at a policy level. The rationale
and controversy of these approaches are discussed
in this article. The authors suggest that all units
treating mechanically ventilated patients should
have a ventilator-associated pneumonia prevention
protocol in place, and ventilator-associated
pneumonia should be seriously considered as a key
performance indicator in local intensive care units.
Introduction
Ventilator-associated pneumonia (VAP) is the
commonest, yet mostly preventable, infection in
mechanically ventilated patients. It contributes
to increased morbidity, mortality, and total
hospitalisation cost. The estimated overall
attributable mortality of VAP was 13%,1 and 52%
of VAP was preventable.2 The VAP rate should
be expressed as the number of VAP days per 1000
ventilator days to take into account the device-utilisation
duration for meaningful comparison.
From the reports of the National Health and Safety
Network (NHSN) of the US Centers for Disease
Control and Prevention (CDC),3 a decreasing
trend for VAP has been noted in recent years. For
example, from 2002 to 2012, for a mixed medical-surgical
intensive care unit (ICU), the rate decreased
from 5.1 to 0.9 days per 1000 ventilator days. The
highest rates were reported for trauma (from 15.2 to
3.6 days) and burns (from 12.0 to 4.4 days) centres,
while neurological and neurosurgical units reported
intermediate rates (around 2 to 4 days). European
rates are mostly higher, even if the same prevention
strategies are used, and some possible reasons are
explained below.4
Unlike many other conditions for which
definitive diagnoses can be made pre-mortem, the
gold standard of VAP diagnosis can only be made
post-mortem. Clinicians diagnose VAP by radiology,
signs and symptoms, with various methods of
non-invasive and invasive microbiology sampling
(tracheal aspirate, directed or blind broncho-alveolar
lavage, protected specimen brush for qualitative
and/or quantitative culture), and histology.
However, when clinical criteria with microbiology
were compared with autopsy findings, both the
sensitivity and specificity were approximately 70%.5
Moreover, there has been no convincing evidence
that qualitative culture of non-invasive samples,
when compared with quantitative culture of invasive
samples, produced any significant differences in
clinical outcomes.6 In a survey of 27 ICUs of nine
European countries, bronchoscopy was performed
only in 23.3% of episodes of nosocomial pneumonia.7
Bronchoscopy might be considered more for
immunocompromised hosts, both for detection of
atypical organisms (if any) and to exclude infection.
The definition of VAP is therefore inherently
subjective and, hence, contentious. The criteria used,
interobserver variability, and subjectivity in chest
X-ray interpretation affect the VAP rates by almost
two-fold.8 9 All these factors influence findings in preventive trials and hamper comparisons. For surveillance purposes, most institutions use
the surveillance definitions of radiology/signs/symptoms to monitor the rates. Commonly used
surveillance definitions are those of the American
Thoracic Society and Infectious Diseases Society
of America (ATS/IDSA)10 and the CDC NHSN.
The two sets of definitions are similar; the notable
difference being that the ATS/IDSA guidelines
exclude pneumonias occurring in the first 48 hours
after intubation, whereas the CDC NHSN definition
includes pneumonias in the first 48 hours; therefore,
using the latter definition will result in a higher VAP
incidence.
In an attempt to overcome the VAP definition’s
inherent subjectivity, in 2014, the CDC NHSN
started surveillance using the Ventilator-Associated
Event protocol,11 under which a ventilator-associated
condition (VAC) is defined if there are at least 2 days of
stable or decreasing ventilator settings (positive end-expiratory
pressure [PEEP] and fraction of inspired
oxygen) followed by consistently higher settings for
at least 2 days. The sensitivity of the VAC criteria
for the detection of VAP was only 25.9%.12 When
further microbiological and invasive techniques
are employed, a VAC can be further classified into
an infection-related VAC, and possible VAP. This modification enables greater objectivity
and facilitates automated electronic capture, but
captures changes due not only to VAP.
Pathophysiology and its implications for prevention strategies
In terms of pathophysiology, VAP is a misnomer
because its occurrence is not related to the ventilator
per se, but to the presence of the endotracheal tube
(ETT). The ETT allows direct access to the lower
respiratory tract, impairing the cough reflex and
mucociliary clearance, but provides incomplete
sealing to secretions above the cuff. Microaspiration
(of materials from oropharyngeal cavities, sinuses,
gastro-intestinal tract) and biofilm formation
are the two most important mechanisms in VAP
development, while inhalation, bacteraemia and
haematogenous spread play smaller roles. Molecular
analysis showed that most VAP patients had the
same bacteria with the same sequencing in their
oral cavities as in their lungs, and there were even
pathogens found in the lungs that could not be
detected by conventional culture-based methods.13
Types of bacteria vary with the time of onset; in early-onset
VAP, they are commonly Enterobacteriaceae,
Candida albicans and Staphylococcus aureus,14 while
in late-onset VAP, they are Pseudomonas aeruginosa,
Klebsiella pneumoniae, and Escherichia coli.
Evolution of prevention strategies
No single strategy is sufficient to prevent VAP,
and several approaches are necessary. The most
widely practised and well-known set of strategies
is the Ventilator Bundle developed by the Institute
for Healthcare Improvement (IHI) in 2001.15 The
Ventilator Bundle is often mislabelled as the VAP
Bundle, and its original aim was to improve better
ventilator care overall, and not VAP alone, although
its practice does reduce the VAP rate by 45%.15 The
components of the Ventilator Bundle have now
become the core of VAP prevention: (1) elevation
of the head of bed to between 30° and 45°; (2)
daily ‘sedative interruption’ and daily assessment of
readiness to be extubated; (3) peptic ulcer disease
prophylaxis; (4) deep venous thrombosis prophylaxis
(unless contra-indicated); and (5) daily oral care
with chlorhexidine. It is practice of the whole
‘bundle’ that decreases VAP, not just the individual
components. Some components are not directly
related to VAP, namely, deep venous thrombosis and
peptic ulcer disease prophylaxis. Although specific
data on VAP were not available, in general, the risk
of hospital-acquired pneumonia increased when
acid-suppressant medications were used. There
was a slight trend for reduced VAP with sucralfate
compared with a histamine 2 receptor antagonist,
and proton pump inhibitors may be related to a
higher rate of VAP.16 Therefore, acid-suppressant
medications should not be overused. In Hong Kong,
the Centre for Health Protection of the Department
of Health promulgated a set of recommendations of
VAP prevention in 2010.17 In this article, we review
the latest literature regarding VAP prevention, and
the strategies are summarised in the Table. The
rationale and controversy are elaborated in the
following discussion.
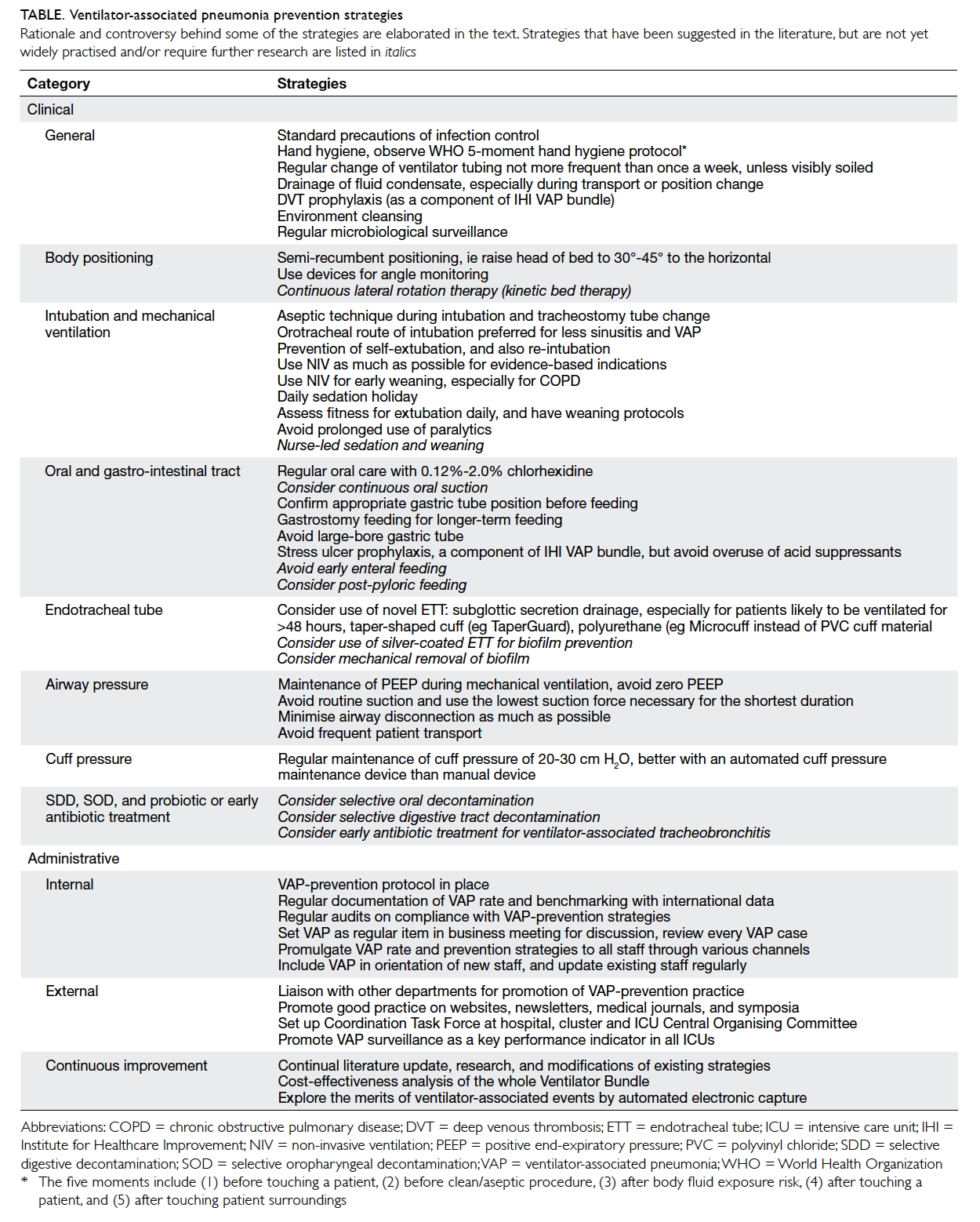
Table. Ventilator-associated pneumonia prevention strategies
Rationale and controversy behind some of the strategies are elaborated in the text. Strategies that have been suggested in the literature, but are not yet widely practised and/or require further research are listed in italics
Prevention strategies
Clinical strategies
General
General principles of infection control should be
followed. Ventilator tubing should not be changed
more frequently than every 1 week unless it is visibly
soiled. In an early prospective randomised trial of
447 patients, decreasing the frequency of ventilator
circuit changes from 3 times to once per week
had no adverse effect on the overall rate of VAP.18
Subsequent trials also showed that circuit change
intervals of 7 and 30 days had even lower risks for
VAP than 2-day intervals.19 There was evidence that
chlorhexidine bathing and hand hygiene compliance
reduced global and specific infection rates, including
VAP.20 A protocol for microbiological surveillance
could be considered to aid in selection of empirical
therapy.
Body positioning
The semi-recumbent position (30°-45° to the
horizontal) is widely practised as one of the
components of the IHI Ventilator Bundle, but which
was based on only one randomised study with a
non–intention-to-treat protocol of 86 mechanically
ventilated patients, comparing the supine and semi-recumbent
positions, in which the VAP rates were
34% and 8%, respectively.21 Subsequent studies were
not able to reproduce these results, and found that a
45° position was difficult to maintain, and the mean
angle achievable was only 28°. It has been suggested
that avoidance of the supine position was beneficial
as, while the semi-recumbent position might reduce
gastro-oropharyngeal aspiration, the effect of
gravity increases hydrostatic pressure of secretions
pooled above the cuff. Recent animal studies found
that mucus flow reversed towards the lungs in the
semi-recumbent position, but drained out in the
horizontal position. In human beings, the trachea/ETT axis is below the horizontal in the lateral
Trendelenburg position (ie lying lateral, at 5°-10°
below the horizontal). The Gravity VAP-Trial,22 an
international randomised controlled trial (RCT)
aiming at enrolment of 800 patients, is ongoing to
compare the efficacy and safety of the two body
positions, namely, the lateral Trendelenburg versus
the semi-recumbent positions, in reducing the
incidence of VAP. The estimated study completion
date is December 2016. Continuous lateral rotation therapy, or kinetic bed therapy, ie lateral rotation of patient to ≥40° on one side (and 80° total arc) using specially designed beds, has been reported to reduce VAP, but was associated with other side-effects
such as intolerance to rotation, unplanned
extubation, loss of vascular access, and arrhythmias.23
Intubation and mechanical ventilation
Non-invasive ventilation (NIV) should be used to
avoid the need for invasive ventilation and also
shorten its duration. Non-invasive ventilation
is particularly beneficial in chronic obstructive
pulmonary disease (COPD), immunocompromised
patients, and acute pulmonary oedema, and it allows
early weaning from invasive ventilation in COPD.
Summary estimates from 16 trials of moderate-to-good quality that included predominantly
participants with COPD suggested that a weaning
strategy that included NIV might reduce mortality
and VAP rates without increasing the risk of weaning
failure or reintubation.24
In a RCT of 128 adult mechanically ventilated
patients, daily interruption of sedation resulted
in a highly significant reduction in time spent on
mechanical ventilation.25 The same authors also
showed in the Awakening and Breathing Controlled
trial that paired daily spontaneous awakening tests
(ie interruption of sedatives) with daily spontaneous
breathing tests resulted in better outcomes for
mechanically ventilated patients.26 Although there is
persistent concern of increased complications with
this practice such as self-extubation, subsequent
study found that this was not the case. A nurse-implemented
sedation protocol has been shown to
decrease VAP.27 Our ICU is also performing a nurse-led
early weaning trial to aim at shortening the
intubation period.
Oral and gastro-intestinal tract
Using chlorhexidine mouth rinse or gel as part of
oral hygiene care, compared with placebo or usual
care, was associated with a reduction in VAP, with
a number needed to treat of 15 (95% confidence
interval, 10-34).28 There is no consensus for the
best practice for oral hygiene care. The optimal
concentration of chlorhexidine solution to use
is not known. Chlorhexidine has been studied in
two strengths: 0.12% and 0.2%, and the US Food
and Drug Administration recommends 0.12% oral
chlorhexidine for use as mouth rinse. There is no
evidence that the following is necessarily better than
using chlorhexidine alone: addition of manual or
powered tooth brushing, using Listerine (Johnson
& Johnson Healthcare Products, New Brunswick
[NJ], US), sodium bicarbonate oral rinses, or use of
povidone-iodine solution. Povidone-iodine seemed
to increase the rate of acute respiratory distress
syndrome.29 A local study showed that continuous
clearance of oral secretion by the saliva ejector might
reduce the rate of VAP, decreasing the duration
of mechanical ventilation, and shortening the
duration of stay of patients in the ICU.30 Although
gastroesophageal aspiration is implicated in VAP
development, in an intention-to-treat study of 449
patients on mechanical ventilation, the absence
of gastric volume monitoring was not inferior to
routine residual gastric volume monitoring in terms
of development of VAP (16.7% in the intervention
group and 15.8% in the control group), nor were there
any significant between-group differences in other
ICU-acquired infections, mechanical ventilation
duration, ICU stay duration, or mortality.31 There
were more VAPs with early enteral feeding, and
less with post-pyloric feeding. Further studies are
required to confirm this.
Endotracheal tube
Novel designs of ETTs target two major mechanisms
of VAP, namely, microaspiration and biofilm
formation.32 33 Microaspiration is controlled by incorporating changes in cuff shape, material, and
addition of a subglottic suction port. Prevention of
biofilm formation is managed by addition of coating,
surface modification, and removal of biofilm by
mechanical means. The conventional ETT cuff (eg
Portex; Smiths Medical International Ltd, Ashford,
UK) is made of polyvinyl chloride (PVC), of which,
when inflated, redundant parts fold on themselves
and, because of the thickness of the material (>50 µ),
microchannels form through which leakage is
possible. By modification of the shape from conical
to tapered (eg TaperGuard; Covidien, Irvine [CA],
US), better contact of the cuff with the trachea
can be achieved, and microchannel formation is
reduced. By modification of the material from
PVC to the thin 7-µ thick polyurethane (eg
Microcuff; Kimberly-Clark Health Care, Roswell
[GA], US), microchannel formation can almost be
prevented. Our benchtop study showed that the
Microcuff consistently outperformed the Portex
and the TaperGuard in terms of leakage, especially
during zero PEEP mechanical ventilation, laboured
breathing, disconnection, and airway suction.34 One
disadvantage of the Microcuff is that the material is
so thin that even moisture diffuses across, leading
to fluid accumulation inside the cuff with resultant
blockage of the pilot balloon tube. This can be solved
by purging the tube with small amount of air. Other
materials being studied are Lycra fibre (Invista,
Wichita [KS], US), silicone, and latex. Addition of
a subglottic suction drainage (SSD) port removes
secretions collected above the cuff (eg TaperGuard
Evac, Covidien). In a meta-analysis of 10 RCTs
with 2213 patients, SSD significantly reduced the
incidence of VAP and early-onset VAP, shortened
ventilation duration by 1.55 days, and prolonged
time to VAP by 3.90 days.35 Ports of SSD are now
available in tracheostomy tubes. One study of such
tracheostomy tubes showed that the prevalences of
VAP were 56% in the control group and 11% in the
suction tracheostomy group.36 The SSD port can be
put into intermittent or continuous suction, with
similar efficacy of VAP prevention. However, the
port could become blocked secondary to suctioned
tracheal mucosa in one third of cases, with the
possibility of tracheal injury.
Of the various coatings of ETT studied, so far,
only silver-based coating (eg Agento IC; Bard Medical,
Covington [GA], US) has been tested in clinical
trials. A meta-analysis identified two high-quality
RCTs with a total of 1630 participants, and showed
that compared with non-coated ETTs, silver-coated
ETTs resulted in a lower incidence of VAP, device-related
adverse events, and microbiological burden,
with no significant difference in total mortality.37
Recently, an ETT with a newly engineered micro-pattern
surface was found to reduce colonisation and
biofilm formation of key VAP-associated pathogens
in vitro by 99.9% compared with unpatterned control
ETTs, including methicillin-resistant S aureus
and P aeruginosa biofilm formation.38 Another
modification of the ETT is by addition of mucus
slurping holes near the distal end of the ETT to keep
the inner walls of the ETT free of mucus deposits.
Attempts are also being made to develop mechanical
means of removing adherent ETT secretions (eg
endOclear catheter; endOclear LLC, Petoskey
[MI], US), which could even relieve life-threatening
ETT obstruction. Another example of mechanical
removal is the Mucus Shaver.39
Airway pressure
Maintenance of a positive pressure gradient across
the ETT cuff is an important factor to prevent
secretions collecting above the cuff to trickle down
across the cuff-trachea interface due to gravity.
This pressure gradient is positive during positive
pressure ventilation, but becomes zero during
airway disconnection, and periodically negative
during laboured inspiration through a narrow
ETT or during airway suction. Without positive
pressure, leakage around the cuff is significant,
especially if the conventional PVC cuff is used and
the cuff pressure is less than 30 cm H2O.34 During
routine care, disconnections of the airway circuit are
frequent, for instance, use of another ventilator for
patient transport, change of the heat-and-moisture
exchanger, spontaneous breathing trials, and
change of ventilator tubing. Such disconnections
should be avoided as far as possible. Spontaneous
breathing trials should not be unduly prolonged
without the intention for resumption of positive
pressure ventilation or direct extubation. There is no evidence that the two methods of humidification, namely, using either a heated humidifier or a heat-and-moisture exchanger, affect the VAP rate.
Cuff pressure
A polyurethane cuff allows for a much lower sealing
pressure than the conventional PVC cuff.34 Periodical
manual checking of cuff pressure maintains adequate
cuff pressure, but the benefit could be offset if the cuff
pressure is accidentally released during checking.
To overcome this issue, a continuous cuff pressure
maintenance device can be used (Fig). A disposable
device called the TRACOE Smart Cuff Manager
(TRACOE Medical GmbH, Nieder-Olm, Germany; Fig)
can also provide the similar functions of being both
a pressure maintenance device (at 30 cm H2O) and
a visual indicator of the adequacy of cuff pressure.
In the largest study to date on the incidence of VAP
comparing a continuous and an intermittent cuff
pressure control system on 284 patients, a lower
incidence of VAP was found (22.0% vs 11.2%).40
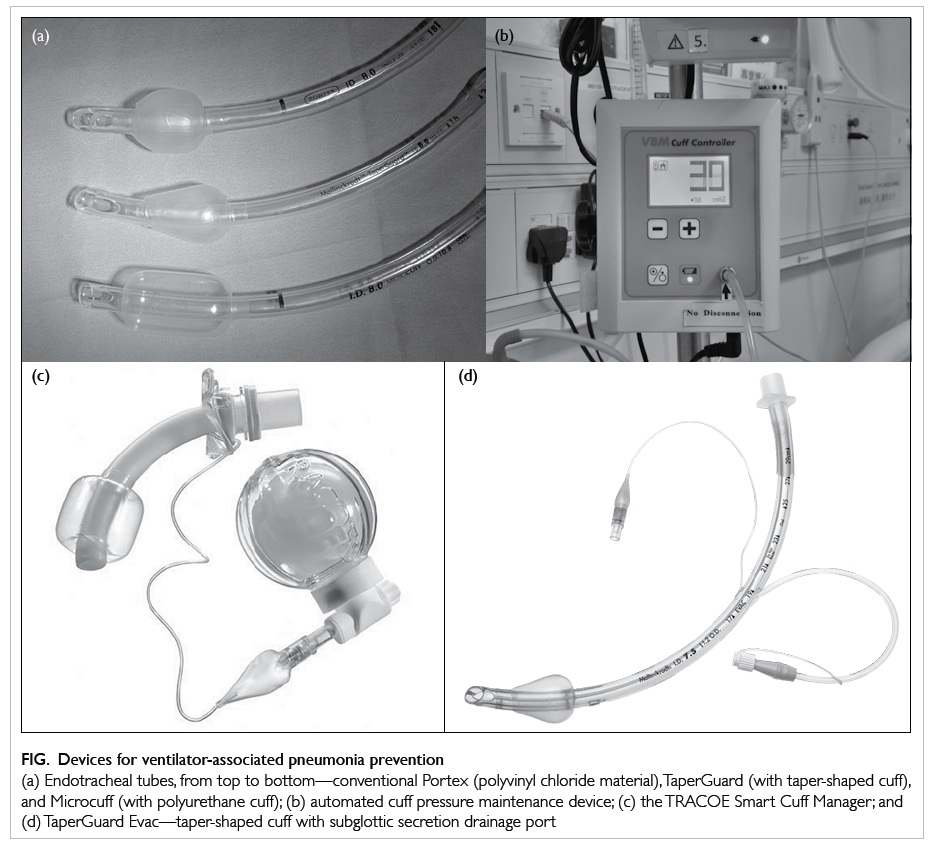
Figure. Devices for ventilator-associated pneumonia prevention
(a) Endotracheal tubes, from top to bottom—conventional Portex (polyvinyl chloride material), TaperGuard (with taper-shaped cuff), and Microcuff (with polyurethane cuff); (b) automated cuff pressure maintenance device; (c) the TRACOE Smart Cuff Manager; and (d) TaperGuard Evac—taper-shaped cuff with subglottic secretion drainage port
Selective digestive decontamination, selective oropharyngeal decontamination, and probiotic or
early antibiotic treatment
Most meta-analyses have shown reductions in VAP
with the use of selective digestive decontamination
or selective oropharyngeal decontamination,
but these interventions are still not being widely
implemented because of concerns of emergence
of antimicrobial resistance in pathogens. A recent
systematic review of 64 studies suggested that such
perceived risk of long-term harm could not be
justified by the available data, but admitted that the
effect on ICU-level antimicrobial resistance rates
was understudied.41 Further studies are also required
for the use of probiotics regarding efficacy and the
risk of colonisation or infection with probiotics.
Around 10% to 30% of ventilator-associated
tracheobronchitis may progress to VAP, and
appropriate antibiotic treatment was independently
associated with reduced risk for transition.42 For
antibiotic prophylaxis, a prospective cohort study
of 129 comatose patients (Glasgow Coma Score ≤8) showed that a single dose of antibiotic within
4 hours of intubation might lower the incidence of
early-onset VAP, with no difference in late-onset
VAP.43 Further RCTs are needed.
Administrative strategies and coordination
Good administrative strategies and coordination
are especially important for the implementation of
this bundle of strategies all the way from patient
care, equipment, infection control, and antibiotic
stewardship. Considering the situation in Hong
Kong that mechanically ventilated patients are
being looked after not only in the ICU, but also in
various critical care areas such as high dependency
units, respiratory wards, and even general wards,
it is imperative that a VAP protocol is in place and
diligently adhered to in every such unit, with regular
audits to ensure compliance. The existence of
written standards for management of mechanically
ventilated patients and the availability of VAP
surveillance systems were positively associated
with compliance with VAP-prevention measures
and should be fostered at a policy level.45 Cost-effectiveness
data for the whole Ventilator Bundle
are scarce and such analysis should be performed
in future research. In one Danish cost-effectiveness
analysis, implementation of the Ventilator Bundle
was found to be potentially cost-effective from
the perspective of the hospital, using outcomes of
prevention of VAP and prevention of death.46 In view
of the necessity for multidisciplinary involvement,
VAP surveillance is better coordinated at a higher
administrative level. The Hong Kong East Cluster of
Hospitals has established a Task Force on Prevention
of VAP in Critical Care Areas since 2013. Ventilator-associated
pneumonia should also be seriously
considered as a key performance indicator in Hong
Kong ICUs, as in other advanced countries.
Conclusion
Ventilator-associated pneumonia is the commonest,
yet mostly preventable, cause of morbidity and
mortality in mechanically ventilated patients.
Successful control of VAP can save total
hospitalisation cost, and is possible by using a multidisciplinary clinical and administrative
approach. All units taking care of mechanically
ventilated patients should have a VAP-prevention
protocol in place, and VAP should be seriously
considered to become a key performance indicator
in local ICUs.
References
1. Melsen WG, Rovers MM, Groenwold RH, et al. Attributable
mortality of ventilator-associated pneumonia: a meta-analysis
of individual patient data from randomised
prevention studies. Lancet Infect Dis 2013;13:665-71. CrossRef
2. Lambert ML, Silversmit G, Savey A, et al. Preventable
proportion of severe infections acquired in intensive
care units: case-mix adjusted estimations from patient-based
surveillance data. Infect Control Hosp Epidemiol
2014;35:494-501. CrossRef
3. Ventilator-associated pneumonia (VAP). Available from:
http://www.cdc.gov/hai/vap/vap.html. Accessed 21 Jul
2014.
4. Kalanuria AA, Zai W, Mirski M. Ventilator-associated pneumonia in the ICU. Crit Care 2014;18:208. CrossRef
5. Fàbregas N, Ewig S, Torres A, et al. Clinical diagnosis of
ventilator associated pneumonia revisited: comparative
validation using immediate post-mortem lung biopsies.
Thorax 1999;54:867-73. CrossRef
6. Berton DC, Kalil AC, Teixeira PJ. Quantitative versus
qualitative cultures of respiratory secretions for clinical
outcomes in patients with ventilator-associated pneumonia.
Cochrane Database Syst Rev 2012;(10):CD006482.
7. Koulenti D, Lisboa T, Brun-Buisson C, et al. Spectrum
of practice in the diagnosis of nosocomial pneumonia in
patients requiring mechanical ventilation in European
intensive care units. Crit Care Med 2009;37:2360-8. CrossRef
8. Novosel TJ, Hodge LA, Weireter LJ, et al. Ventilator-associated
pneumonia: depends on your definition. Am
Surg 2012;78:851-4.
9. Klompas M. Interobserver variability in ventilator-associated
pneumonia surveillance. Am J Infect Control
2010;38:237-9. CrossRef
10. American Thoracic Society; Infectious Diseases Society of
America. Guidelines for the management of adults with
hospital-acquired, ventilator-associated, and healthcare-associated
pneumonia. Am J Respir Crit Care Med
2005;171:388-416. CrossRef
11. Ventilator-Associated Event (VAE). Available from: http://www.cdc.gov/nhsn/PDFs/pscManual/10-VAE_FINAL.pdf. Accessed 1 Jan 2015.
12. Muscedere J, Sinuff T, Heyland DK, et al. The clinical
impact and preventability of ventilator-associated
conditions in critically ill patients who are mechanically
ventilated. Chest 2013;144:1453-60. CrossRef
13. Bahrani-Mougeot FK, Paster BJ, Coleman S, et al.
Molecular analysis of oral and respiratory bacterial species
associated with ventilator-associated pneumonia. J Clin
Microbiol 2007;45:1588-93. CrossRef
14. Charles MP, Easow JM, Joseph NM, Ravishankar M,
Kumar S, Sivaraman U. Aetiological agents of ventilator-associated
pneumonia and its resistance pattern—a threat
for treatment. Australas Med J 2013;6:430-4. CrossRef
15. How-to guide: Prevent ventilator-associated pneumonia.
Available from: http://www.ihi.org/resources/Pages/Tools/HowtoGuidePreventVAP.aspx. Accessed 20 Jul
2014.
16. Khorvash F, Abbasi S, Meidani M, Dehdashti F, Ataei B.
The comparison between proton pump inhibitors and
sucralfate in incidence of ventilator associated pneumonia
in critically ill patients. Adv Biomed Res 2014;3:52. CrossRef
17. Recommendations on prevention of ventilator-associated
pneumonia. Scientific Committee on Infection Control,
and Infection Control Branch, Centre for Health
Protection, Department of Health; 2010.
18. Long MN, Wickstrom G, Grimes A, Benton CF, Belcher B,
Stamm AM. Prospective, randomized study of ventilator-associated
pneumonia in patients with one versus three
ventilator circuit changes per week. Infect Control Hosp
Epidemiol 1996;17:14-9. CrossRef
19. Fink JB, Krause SA, Barrett L, Schaaff D, Alex CG.
Extending ventilator circuit change interval beyond 2 days
reduces the likelihood of ventilator-associated pneumonia.
Chest 1998;113:405-11. CrossRef
20. Martínez-Reséndez MF, Garza-González E, Mendoza-Olazaran S, et al. Impact of daily chlorhexidine baths and
hand hygiene compliance on nosocomial infection rates in
critically ill patients. Am J Infect Control 2014;42:713-7. CrossRef
21. Drakulovic MB, Torres A, Bauer TT, Nicolas JM, Nogué
S, Ferrer M. Supine body position as a risk factor for
nosocomial pneumonia in mechanically ventilated
patients: a randomised trial. Lancet 1999;354:1851-8. CrossRef
22. The Gravity VAP network. Gravity VAP-Trial. Available
from: http://compartint.net/gravityvaptrial/joomla/. Accessed 21 Jul 2014.
23. Staudinger T, Bojic A, Holzinger U, et al. Continuous lateral rotation therapy to prevent ventilator-associated pneumonia. Crit Care Med 2010;38:486-90. CrossRef
24. Burns KE, Meade MO, Premji A, Adhikari NK. Noninvasive
positive-pressure ventilation as a weaning strategy for
intubated adults with respiratory failure. Cochrane
Database Syst Rev 2013;(12):CD004127.
25. Kress JP, Pohlman AS, O’Connor MF, Hall JB. Daily
interruption of sedative infusions in critically ill patients
undergoing mechanical ventilation. N Engl J Med
2000;342:1471-7. CrossRef
26. Girard TD, Kress JP, Fuchs BD, et al. Efficacy and safety
of a paired sedation and ventilator weaning protocol
for mechanically ventilated patients in intensive care
(Awakening and Breathing Controlled trial): a randomised
controlled trial. Lancet 2008;371:126-34. CrossRef
27. Quenot JP, Ladoire S, Devoucoux F, et al. Effect of a
nurse-implemented sedation protocol on the incidence
of ventilator-associated pneumonia. Crit Care Med
2007;35:2031-6. CrossRef
28. Shi Z, Xie H, Wang P, et al. Oral hygiene care for critically
ill patients to prevent ventilator-associated pneumonia.
Cochrane Database Syst Rev 2013;(8):CD008367.
29. Seguin P, Laviolle B, Dahyot-Fizelier C, et al. Effect of
oropharyngeal povidone-iodine preventive oral care
on ventilator-associated pneumonia in severely brain-injured
or cerebral hemorrhage patients: a multicenter,
randomized controlled trial. Crit Care Med 2014;42:1-8. CrossRef
30. Chow MC, Kwok SM, Luk HW, Law JW, Leung BP. Effect
of continuous oral suctioning on the development of
ventilator-associated pneumonia: a pilot randomized
controlled trial. Int J Nurs Stud 2012;49:1333-41. CrossRef
31. Reignier J, Mercier E, Le Gouge A, et al. Effect of not
monitoring residual gastric volume on risk of ventilator-associated
pneumonia in adults receiving mechanical
ventilation and early enteral feeding: a randomized
controlled trial. JAMA 2013;309:249-56. CrossRef
32. Fernandez JF, Levine SM, Restrepo MI. Technologic
advances in endotracheal tubes for prevention of ventilator-associated
pneumonia. Chest 2012;142:231-8. CrossRef
33. Lam SM, Lau AC. Prevention of ventilator-associated
pneumonia (VAP) by novel endotracheal tube designs.
Hong Kong Lung Foundation, Hong Kong Thoracic Society
& ACCP (HK & Macau Chapter) Newsletter 2011: 32-5.
34. Lau AC, Lam SM, Yan WW. Benchtop study of leakages
across the Portex, TaperGuard, and Microcuff endotracheal
tubes under simulated clinical conditions. Hong Kong Med
J 2014;20:7-15. CrossRef
35. Wang F, Bo L, Tang L, et al. Subglottic secretion drainage
for preventing ventilator-associated pneumonia: an
updated meta-analysis of randomized controlled trials. J
Trauma Acute Care Surg 2012;72:1276-85.
36. Ledgerwood LG, Salgado MD, Black H, Yoneda K, Sievers
A, Belafsky PC. Tracheotomy tubes with suction above the
cuff reduce the rate of ventilator-associated pneumonia
in intensive care unit patients. Ann Otol Rhinol Laryngol
2013;122:3-8. CrossRef
37. Li X, Yuan Q, Wang L, Du L, Deng L. Silver-coated
endotracheal tube versus non-coated endotracheal tube
for preventing ventilator-associated pneumonia among
adults: a systematic review of randomized controlled trials.
J Evid Based Med 2012;5:25-30. CrossRef
38. May RM, Hoffman MG, Sogo MJ, et al. Micro-patterned
surfaces reduce bacterial colonization and biofilm
formation in vitro: potential for enhancing endotracheal
tube designs. Clin Transl Med 2014;3:8. CrossRef
39. Berra L, Coppadoro A, Bittner EA, et al. A clinical
assessment of the Mucus Shaver: a device to keep the
endotracheal tube free from secretions. Crit Care Med
2012;40:119-24. CrossRef
40. Lorente L, Lecuona M, Jiménez A, et al. Continuous
endotracheal tube cuff pressure control system protects
against ventilator-associated pneumonia. Crit Care
2014;18:R77. CrossRef
41. Daneman N, Sarwar S, Fowler RA, Cuthbertson BH;
SuDDICU Canadian Study Group. Effect of selective
decontamination on antimicrobial resistance in intensive
care units: a systematic review and meta-analysis. Lancet
Infect Dis 2013;13:328-41. CrossRef
42. Nseir S, Martin-Loeches I, Makris D, et al. Impact of
appropriate antimicrobial treatment on transition from
ventilator-associated tracheobronchitis to ventilator-associated
pneumonia. Crit Care 2014;18:R129. CrossRef
43. Vallés J, Peredo R, Burgueño MJ, et al. Efficacy of single-dose antibiotic against early-onset pneumonia in comatose
patients who are ventilated. Chest 2013;143:1219-25. CrossRef
44. Kaier K, Lambert ML, Frank UK, et al. Impact of availability
of guidelines and active surveillance in reducing the
incidence of ventilator-associated pneumonia in Europe
and worldwide. BMC Infect Dis 2014;14:199. CrossRef
45. Møller AH, Hansen L, Jensen MS, Ehlers LH. A cost-effectiveness
analysis of reducing ventilator-associated
pneumonia at a Danish ICU with ventilator bundle. J Med
Econ 2012;15:285-92. CrossRef
Find HKMJ in MEDLINE: