Hong Kong Med J 2014;20:7–15 | Number 1, February 2014 | Epub 22 Jul 2013
DOI: 10.12809/hkmj133930
© Hong Kong Academy of Medicine. CC BY-NC-ND 4.0
ORIGINAL ARTICLE
Benchtop study of leakages across the Portex, TaperGuard, and Microcuff endotracheal tubes under simulated clinical conditions
Arthur CW Lau, FHKAM (Medicine);
SM Lam, FHKAM (Medicine);
WW Yan, FHKAM (Medicine)
Department of Intensive Care, Pamela Youde Nethersole Eastern Hospital, Chai Wan, Hong Kong
Corresponding author: Dr ACW Lau (laucw3@ha.org.hk)
Abstract
Objectives: To compare three endotracheal tubes for leakage across the
cuff (microaspiration) under a comprehensive set of simulated
clinical situations. These were the Mallinckrodt TaperGuard
(Covidien, US) with a tapered polyvinyl chloride cuff; the
KimVent Microcuff (Kimberly-Clark Health Care, US) with a
cylindrical polyurethane cuff; and a conventional Portex (Smiths
Medical International Ltd, UK) with a globular polyvinyl chloride
cuff.
Design: A benchtop experimental study.
Setting and materials: A silicone cylinder serving as the model trachea was intubated
with each of the three endotracheal tubes, one at a time. A total of
20 mL of water were added above the cuff and leakage measured
every minute for 20 minutes under five simulated mechanical
ventilation scenarios, including different positive end-expiratory
pressure levels, and disconnection with and without spontaneous
breathing efforts. Each scenario was studied under three cuff
pressures of 10, 20 and 30 cm H2O, and then repeated with the
application of a continuous suction force of 200 cm H2O, and
leakage measured every minute for 3 minutes.
Results: The outcome of interest was the cumulative amount of
leakage. The Microcuff endotracheal tubes with an ultrathin
polyurethane cuff consistently provided the best protection
against microaspiration under all simulated clinical situations,
followed by TaperGuard with a tapered cuff, and lastly Portex
with a globular polyvinyl chloride cuff. Clinical scenarios
associated with the greatest leakage were mechanical
ventilation with zero positive end-expiratory pressure, circuit
disconnection with spontaneous breathing efforts, application
of suction, and a low cuff pressure.
Conclusions: Microcuff endotracheal tubes outperformed TaperGuard and
Portex endotracheal tubes in preventing microaspiration,
which is one of the major mechanisms for ventilator-associated
pneumonia.
New knowledge added by this
study
- Microcuff endotracheal tubes (ETTs) with an ultrathin polyurethane cylindrical cuff provided the best protection against microaspiration under diverse situations.
- TaperGuard ETT with a tapered polyvinyl chloride (PVC) cuff provided protection against microaspiration in simulated at-risk situations, given that the cuff pressure was maintained at the recommended 20 to 30 cm of H2O.
- The most widely used Portex ETT with a globular PVC cuff did not protect against microaspiration under these at-risk simulated situations, even at recommended cuff pressures of 20 to 30 cm H2O.
- This study supports more widespread use of ETTs with an ultrathin polyurethane cuff (eg the Microcuff) to better prevent microaspiration, which is one of the major mechanisms of ventilator-associated pneumonia.
- Some scenarios appear more prone to microaspirations, eg zero positive end-expiratory pressure, total disconnection, and spontaneous breathing. The Microcuff ETT outperformed other ETTs, particularly in such scenarios.
Introduction
One of the major mechanisms of ventilator-associated
pneumonia (VAP) is microaspiration of bacteria-colonised
oropharyngeal secretions that collect
above the inflated cuff of the endotracheal tube (ETT).
In Hong Kong, for several decades, the cuff of the
most commonly used ETT has been made of polyvinyl
chloride (PVC) and has a globular shape. This type of
cuff protects against microaspiration poorly, due to
microchannels formed from infolding of redundant
cuff material after inflation.1 Novel designs of the ETT
cuff attempt to overcome this problem by modifying
the material from the thicker (50- to 80-micron) PVC
to the ultrathin (10-micron) polyurethane (PU),2 and
the cuff shape from globular to tapered or cylindrical.
In addition, a subglottic secretion drainage port for
aspiration of secretions collected above the cuff is
available in some ETTs. Although these novel ETTs
have been available for many years,3 4 they are not
widely used locally. Reasons include inadequate
cost-effectiveness data, even though most studies
reported favourable efficacy in reducing VAP, though not necessarily mortality.1
Many previous benchtop studies have shown
the benefits of these novel designs, but mostly under
a limited number of conditions, or under a static
condition without the dynamic effect of different
levels of positive pressures from mechanical
ventilation or negative pressures associated with
spontaneous breathing and/or suction.5 6 Moreover,
they mostly reported the amount of leakage at a
particular time point without showing continuous
trends.5 The aim of the present study was to compare
these novel ETTs under a comprehensive set of
simulated clinical conditions, and to find the bestperforming
tube in which downward leakage of fluid
across the cuff was minimal.
Methods
The experimental setup is shown in Figure 1. The
three types of ETTs under test were the Portex
Endotracheal Tube (Smiths Medical International
Ltd, UK) with a globular PVC cuff; the Mallinckrodt
TaperGuard Endotracheal Tube (Covidien, US) with
a tapered PVC cuff; and the KimVent Microcuff
Endotracheal Tube (Kimberly-Clark Health Care, US)
with a cylindrical PU cuff (Fig 2). A transparent, hollow
silicone cylinder of length 20 cm and an internal
diameter of 2 cm was used as the model trachea. An
internal diameter of 2 cm was chosen because from
autopsy studies, the mean diameters of male and
female tracheas were 2.2 cm and 1.8 cm, respectively.7
A flexible and extensible tube was added to the
proximal end of the model trachea to prevent fluid
from splashing out where significant upward leakage
results from high positive ventilatory pressure.5 The
ETT under study was connected to a SERVO-i Adult
ventilator (Maquet GmbH & Co. KG, Germany). The model trachea was inclined at 35 degrees to
the horizontal to simulate the semi-recumbent
position for VAP prevention. Cuff pressure (Pcuff)
was maintained by an automated maintenance setup
as devised and modified from a previous study.8 In
short, compressed air in the range of 2 to 3 L/min
was used to inflate the cuff and the pressure was
altered with a leakage port along the circuit, to
maintain the desired Pcuff within a range of ± 1 cm
H2O at end-expiration. The PVC cuff was monitored
continuously using a calibrated electronic pressure
transducer (Model HCLA0050EU; Sensortechnics
GmbH, Germany), with signals digitally transformed
by an analogue-to-digital converter (NI USB-6212;
National Instruments, US), so as to display on a
computer using the LabVIEW 2010 software (National
Instruments Corporation, US). The proximal end of a
Y-piece was connected to the distal end of the model
trachea. To the Y-piece’s vertical end, a water trap
made of a 20-mL syringe was connected. For the study
of positive pressure mechanical ventilation, it was
connected to a lung simulator (SMS Lung Simulator;
SMS Technologies, UK) at its distal end. For the study
of spontaneous breathing, it was connected to a
Huszczuk-Whipp-Wasserman Gas Exchange System
Validator (MedGraphics, US)9 which generated
breaths to mimic different metabolic rates; and for
the study of total disconnection, it was opened to
atmospheric pressure.
The scenarios simulated are shown in Table 1.
Clear water (20 mL) was added above the ETT cuff. The
whole process was recorded by a video recorder and
leakage was measured as observed in the syringe for 20 minutes. Each scenario was studied under different
Pcuffs of 10, 20, and 30 cm H2O. For each scenario
at each Pcuff, two tubes of the same ETT type were
tested, and each tube was studied repeatedly for 4
times, therefore making a total of 8 measurements for
each ETT type per scenario and Pcuff.
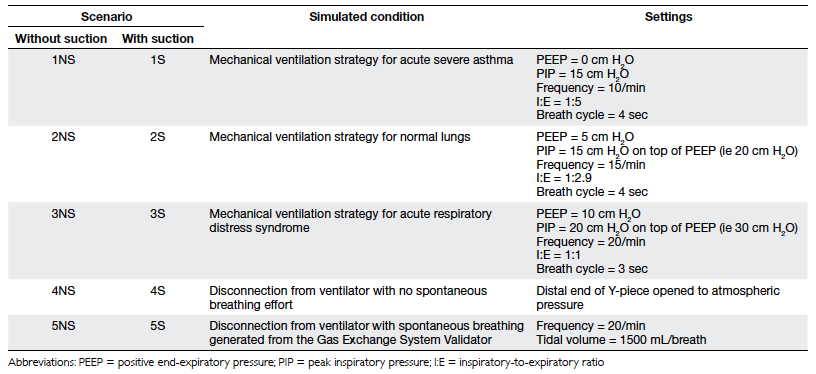
Table 1. Simulated clinical scenarios tested under three different cuff pressures (10, 20, and 30 cm H2O) using the three types of endotracheal tubes (ETTs)
The same scenarios were then repeated under
sustained tracheal suction by placing a suction
catheter (12-Fr closed suction catheter) inside the
ETT near the Murphy eye,10 and a suction pressure of
200 cm H2O was applied continuously for 3 minutes.
The primary measurement was the downward
leakage across the cuff, defined as the amount of
fluid collected in the syringe (the fluid trap) every
minute during the observation period.
Statistical analysis
Between- and within-group analysis of variance
(ANOVA) was used for analysis of the amount of
downward leakage during the whole observation
period, with the aim of comparing the difference
in leakage between the three types of ETTs for each
scenario. Each scenario at each Pcuff was analysed
separately, in which between-group data were different types of ETTs and within-group data were
the cumulative amount (in mL) of leakage over each
observation period. Statistical analysis was performed
using IBM SPSS software version 20 (SPSS, Inc, IBM,
US).
Results
Results of the five scenarios without suction (1NS to
5NS) are shown in Figure 3. The P values represent
analyses by ANOVA of the cumulative leakage
over the whole 20-minute observation period. The
cumulative leakages at the 20-minute time point
are shown in Table 2. In summary, in scenario 1NS (positive end-expiratory pressure [PEEP] of 0 cm H2O),
the Microcuff outperformed the other two ETTs at all
Pcuffs. At a Pcuff of 30 cm H2O, only the Portex showed
leakage, which was early and massive. At a Pcuff of 20
cm H2O, only Microcuff did not leak. At a Pcuff of 10
cm H2O, all three ETTs leaked, but the rate was fastest
in Portex and lowest in Microcuff. In scenario 2NS
(PEEP of 5 cm H2O), at Pcuffs of 30 and 20 cm H2O,
all three ETTs showed no leakage, while at a Pcuff of
10 cm H2O, only Portex showed minimal leakage. In
scenario 3NS (PEEP of 10 cm H2O), none leaked. In
scenario 4NS (disconnection), significant leakage
occurred only in Portex at Pcuffs of 30 and 20 cm H2O.
At a Pcuff of 10 cm H2O, all leaked, but the rate was
lowest with Microcuff. In scenario 5NS (spontaneous
breathing), addition of spontaneous breathing led to
leakage in all ETTs at Pcuffs of 10 and 20 cm H2O, but
the rate remained the lowest in Microcuff. At a Pcuff of
30 cm H2O, only Microcuff showed minimal leakage.
Results of the five scenarios with suction (1S to
5S) are shown in Figure 4. The P values pertained to
analyses by ANOVA of the whole 3-minute observation
period. The cumulative leakages at the 3-minute time
point are shown in Table 2. In summary, in scenario
1S (PEEP of 0 cm H2O), at Pcuffs of 30 and 20 cm H2O,
only Microcuff was protective, while the other two
leaked almost instantly. At a Pcuff of 10 cm H2O, all
three ETTs leaked, but Microcuff leaked very slowly. In
scenario 2S (PEEP of 5 cm H2O), at a Pcuff of 30 cm H2O,
leakage occurred instantly with Portex, in contrast to
zero leakage in the corresponding scenario without
suction. At a Pcuff of 20 cm H2O, only Microcuff did
not leak. At a Pcuff of 10 cm H2O, all three ETTs leaked,
but Microcuff leaked very slowly. In scenario 3S (PEEP
of 10 cm H2O), at a Pcuff of 30 cm H2O, none leaked. At
a Pcuff of 20 cm H2O, minimal leakage occurred with
Portex. In scenario 4S (disconnection), at a Pcuff of 30
cm H2O, significant leakage was found in Portex. At
a Pcuff of 20 cm H2O, only Microcuff was protective
whilst TaperGuard leaked slowly. At a Pcuff of 10
cm H2O, Microcuff was still protective with minimal
leakage at 3 minutes. In scenario 5S (spontaneous
breathing), at a Pcuff of 30 cm H2O, significant leakage
was found in Portex. At a Pcuff of 20 cm H2O, Portex
leaked significantly, TaperGuard leaked very slowly,
while Microcuff was protective. At a Pcuff of 10 cm
H2O, all three leaked.
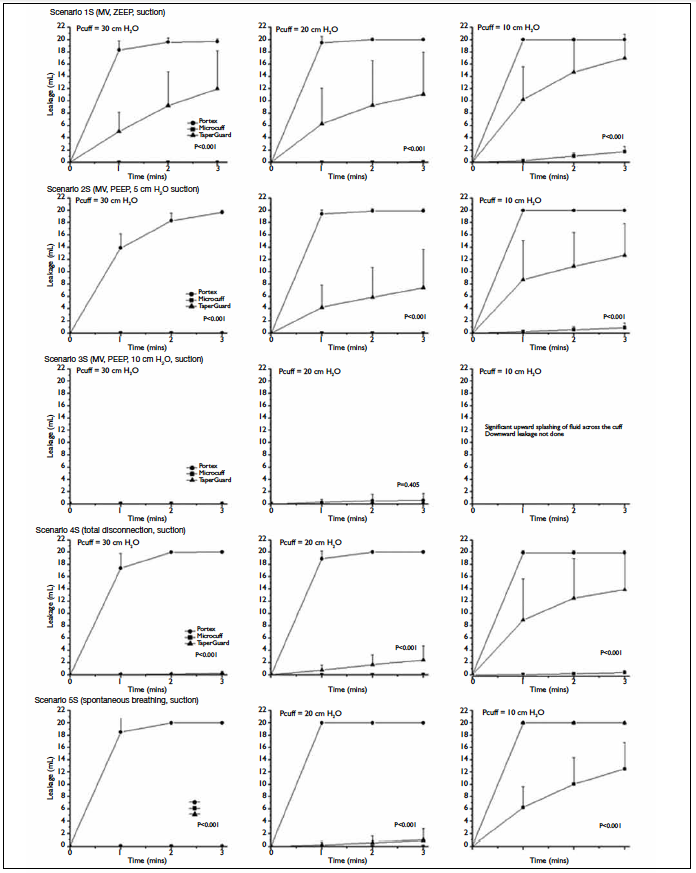
Figure 4. Downward leakage in the model trachea in the five scenarios after application of continuous suction of 200 cm H2O for 3 minutes (1S to 5S)
Discussion
The present benchtop study showed that under
the various simulated scenarios studied (positive
pressure ventilation, disconnection, spontaneous
breathing, with or without the application of suction),
the Microcuff ETT consistently outperformed the
others with the least downward leak and the lowest
sealing pressure, whereas the TaperGuard ETT was in
second place. The Portex ETT performed the worst, with significant leakage whenever there was a loss of
positive airway pressure even at the recommended
Pcuffs of 20 to 30 cm H2O.
Among the limitations of the present study,
many in-vivo factors were not or could not be fully
simulated but might have affected the leakage rate.
In-vivo leakage could be greater when there is a
change in tracheal dimensions during inspiration and/or imperfect conformation of the circular cuff to the
trachea (which could have different anteroposterior
compared with transverse dimensions), and when
there is a sudden change in airway pressure or the
cuff position inside the trachea as the patient coughs
or moves. Moreover, the seal between the cuff and
the moist tracheal mucosa might actually be better,
especially with the use of lubricant at the time of
intubation.4 11 12 The upward sweeping movement of
the ciliated mucosa might also decrease aspiration.
Furthermore, subglottic collections are more viscous
than water, and their volume is not likely to be as
high as 20 mL. This volume was chosen in the current
experiment to better discriminate ETT performance
and minimise measurement errors. Dynamic patient
factors may also affect leakage. For example, leakage
in the first scenario (zero PEEP) might be less if
significant auto-PEEP develops in severe airflow
obstruction, and the size of the cuff relative to the
trachea might increase or decrease leakage. Nor
did we test the effect of subglottic suction, which
is extremely effective in removing fluid collected
above the cuff. However, the effectiveness of
subglottic suction might differ in vivo, depending
on the viscosity of secretions and apposition of
the posterior mucosal wall obstructing the suction
lumen and interfering with such efforts.13 According
to guidelines on endotracheal suction for adults,14
the lowest suction pressure that can effectively clear
secretions should be used, which should not exceed
150 cm H2O, and for not more than 15 seconds. We
deliberately used sustained suction at 200 cm H2O for
3 minutes to better discriminate the performance of
each ETT. Notably though, in the present experiment
it was observed that leakage occurred within the first
second of suction. Although it can be argued that a
20-minute observation period may be too short, we
found that prolonging this period led to excessive
evaporation of the water above the cuff. We did not
use coloured water because we measured water
collected in the syringe, simulating the volume of
fluid actually aspirated into the lower airway, and
not the minute amounts that might just leak and
stay around the cuff. Fluid more viscous than water
was not used because firstly, human secretions can
never be fully simulated, and secondly, should there
be any leakage, fluid of a lower viscosity (like water)
was considered more liable to leak. Furthermore,
the need for thorough cleansing of viscous fluid by
dismantling the connections of the apparatus after each set of experiments was another consideration.
Such cleansing was regarded as not feasible, because
each connection needed to be secured with glue and
tapes to withstand the high positive airway pressure
and this would take an unrealistic amount of time to
do so repeatedly.
The present experiment clearly discriminated
the performance characteristics of the three ETTs.
Infolding of the excess and thick PVC material in the
Portex ETT formed micro-channels through which
leakage occurred. By modifying the cuff to a tapered
shape, leakage in the TaperGuard ETT was minimised,
as there was a point along the length of the cuff where
it fitted the trachea perfectly without the infolding of
excess cuff material. In the Microcuff ETT, infolding
of excess cuff material was still observed. However,
micro-channels were not formed because of the
much thinner PU material. Its cylindrical shape also
provided a larger contact area between the cuff and
tracheal wall, thus providing a better seal than the
other two types of ETTs.
One of the clinical implications of our findings
was that PEEP guards against downward leakage
across cuffs, irrespective of the ETT type and Pcuff. In a
previous study, it was shown that only the application
of a 35 cm H2O PEEP prior to cuff deflation at extubation
was protective against aspiration, but not open or
closed suction (that supposedly removes aspirated
water).15 This protective effect of a higher PEEP was
independent of the peak inspiratory pressure (PIP) as
leakage still occurred in scenario 1NS (with a PIP of 15
cm H2O); such finding was in line with another study
showing that only higher PEEP values and not higher
inspiratory pressures were protective.16 However,
the protective effect of PEEP, especially with low
pressures of around 5 cm of H2O, was counteracted
by the application of suction, as shown by the
appearance of leakage when suction was applied to
the Portex and TaperGuard ETTs as in scenario 2S.
Another study showed that when suction at 200 or
300 mbar was applied via ETTs with a PVC cuff, leakage
could only be reduced by transiently increasing Pcuff
to 50 cm H2O, and not at all by increasing the PEEP
from 5 to 10 cm H2O or the PIP from 15 to 25 cm H2O.17
This same study also showed that the PU cuff almost
eliminated leakage under all suction pressures, and
all PEEP or PIP values.17 Therefore, to prevent leakage
during suction, a Pcuff of 50 cm H2O may be necessary
unless a PU cuff is used, while the suction duration
and force should be reduced to a minimum, and
routine suction should always be avoided. Given that
suctioning results in the loss of PEEP and recruitment
manoeuvres are recommended thereafter,14 it may be
worth studying whether the application of high PEEP
during suction can prevent both derecruitment and
leakage across the cuff.
Similar to the effect of applying no PEEP during
mechanical ventilation, disconnection from such ventilation results in significant leakage across the cuff,
and should be avoided as far as possible. Harnessing
the portability of an intensive care unit ventilator
to avoid circuit disconnections should therefore be
considered when patients are transported. When
disconnection is necessary, its duration should be
kept to a minimum with the maintenance of PEEP.
For example, a PEEP valve from a bag-valve device
may minimise leakage across the cuff. Spontaneous
breathing during disconnection, which creates a
negative intrathoracic pressure, further exacerbates
downward leakage (scenario 5NS). Another study has
also found that leakage increased with increasing
inspiratory effort.16 Therefore, during disconnection
for spontaneous breathing trials, microaspiration is
to be expected if the conventional globular PVC cuff
is used. Even with the use of novel ETTs, a high patient
inspiratory effort during a trial of spontaneous
breathing is conducive to microaspiration and
should be anticipated. Extrapolating these results, a
high patient inspiratory effort while on mechanical
ventilation may prove to be another scenario at high
risk of microaspiration.
Under situations with a high risk of
microaspiration, namely zero or low PEEP, circuit
disconnection, in the presence of high patient
inspiratory effort and application of a suction force,
the type of ETT used will make a difference to the rate
of downward leakage. The Microcuff ETT was shown
to offer the best protection in these situations. The
TaperGuard ETT was protective if a higher Pcuff
could be maintained, and a Pcuff maintenance device
(keeping it between 20 and 30 cm of H2O) may be
helpful. Notably, the most commonly used Portex ETT
provided the least protection against microaspiration,
and leakage occurred in these situations despite
maintaining a Pcuff at the recommended 20 to 30 cm
H2O.
Based on the findings of the present study,
further clinical trials on VAP prevention using novel
ETTs less prone to microaspiration are needed. These
should control for confounding factors including
PEEP, airway suction, use of automated Pcuff
maintenance devices, and airway disconnections. At
the time of writing, the cost of a Microcuff ETT was
around 6 times that of a Portex ETT (HK$68 vs HK$11),
and a TaperGuard ETT was around double (HK$20).
Although cost-effectiveness analysis is worthwhile as
a basis for wider promotion of the novel ETTs, taking
into account the small absolute cost difference, there
may be a case for just switching ETTs to those with a
lesser tendency to leak until evidence to the contrary
appears.
Conclusions
The present benchtop study showed that a higher
PEEP, avoidance of unnecessary circuit disconnections and suctioning, and maintenance of adequate
Pcuff are important in minimising microaspiration.
The Microcuff ETT was shown to be superior to
TaperGuard and Portex ETTs in preventing leakage
across the cuff. As microaspiration is one of the
major mechanisms of VAP, more widespread use of
ETTs with a PU cuff, combined with other prevention measures (eg bedhead elevation, oral hygiene)
may help to reduce the frequency of associated
pneumonias.
Declaration
No conflicts of interest were declared by the authors.
References
1. Fernandez JF, Levine SM, Restrepo MI. Technologic advances
in endotracheal tubes for prevention of ventilator-associated
pneumonia. Chest 2012;142:231-8. Crossref
2. Bulpa P, Bouhon S, Schryvers F, et al. Microaspirations during
mechanical ventilation: polyurethane versus polyvinyl
cuffed endotracheal tubes. Crit Care 2010;14(Suppl
1):228S. Crossref
3. Dullenkopf A, Gerber A, Weiss M. Fluid leakage past tracheal
tube cuffs: evaluation of the new Microcuff endotracheal
tube. Intensive Care Med 2003;29:1849-53. Crossref
4. Dullenkopf A, Schmitz A, Frei M, Gerber AC, Weiss M. Air
leakage around endotracheal tube cuffs. Eur J Anaesthesiol
2004;21:448-53. Crossref
5. Pitts R, Fisher D, Sulemanji D, Kratohvil J, Jiang Y, Kacmarek
R. Variables affecting leakage past endotracheal tube cuffs: a
bench study. Intensive Care Med 2010;36:2066-73. Crossref
6. Zanella A, Scaravilli V, Isgrò S, et al. Fluid leakage across
tracheal tube cuff, effect of different cuff material, shape,
and positive expiratory pressure: a bench-top study.
Intensive Care Med 2011;37:343-7. Crossref
7. Mehta S, Myat HM. The cross-sectional shape and
circumference of the human trachea. Ann R Coll Surg Engl
1984;66:356-8.
8. Farré R, Rotger M, Ferre M, Torres A, Navajas D. Automatic
regulation of the cuff pressure in endotracheally-intubated
patients. Eur Respir J 2002;20:1010-3. Crossref
9. Huszczuk A, Whipp BJ, Wasserman K. A respiratory gas
exchange simulator for routine calibrator in metabolic
studies. Eur Respir J 1990;3:465-8..
10. Dave MH, Frotzler A, Madjdpour C, Koepfer N, Weiss M.
Massive aspiration past the tracheal tube cuff caused by
closed tracheal suction system. J Intensive Care Med 2011
Feb 7. Epub ahead of print. Crossref
11. Dave MH, Koepfer N, Madjdpour C, Frotzler A, Weiss M.
Tracheal fluid leakage in benchtop trials: comparison of
static versus dynamic ventilation model with and without
lubrication. J Anesth 2010;24:247-52. Crossref
12. Blunt MC, Young PJ, Patil A, Haddock A. Gel lubrication
of the tracheal tube cuff reduces pulmonary aspiration.
Anesthesiology 2001;95:377-81. Crossref
13. Dragoumanis CK, Vretzakis GI, Papaioannou VE, Didilis VN,
Vogiatzaki TD, Pneumatikos IA. Investigating the failure to
aspirate subglottic secretions with the Evac endotracheal
tube. Anesth Analg 2007;105:1083-5. Crossref
14. American Association for Respiratory Care. AARC
Clinical Practice Guidelines. Endotracheal suctioning of
mechanically ventilated patients with artificial airways
2010. Respir Care 2010;55:758-64.
15. Hodd J, Doyle A, Carter J, Albarran J, Young P. Increasing
positive end expiratory pressure at extubation reduces
subglottic secretion aspiration in a bench-top model. Nurs
Crit Care 2010;15:257-61. Crossref
16. Ouanes I, Lyazidi A, Danin PE, et al. Mechanical influences
on fluid leakage past the tracheal tube cuff in a benchtop
model. Intensive Care Med 2011;37:695-700. Crossref
17. Dave MH, Frotzler A, Weiss M. Closed tracheal suction and
fluid aspiration past the tracheal tube. Impact of tube cuff
and airway pressure. Minerva Anestesiol 2011;77:166-71.